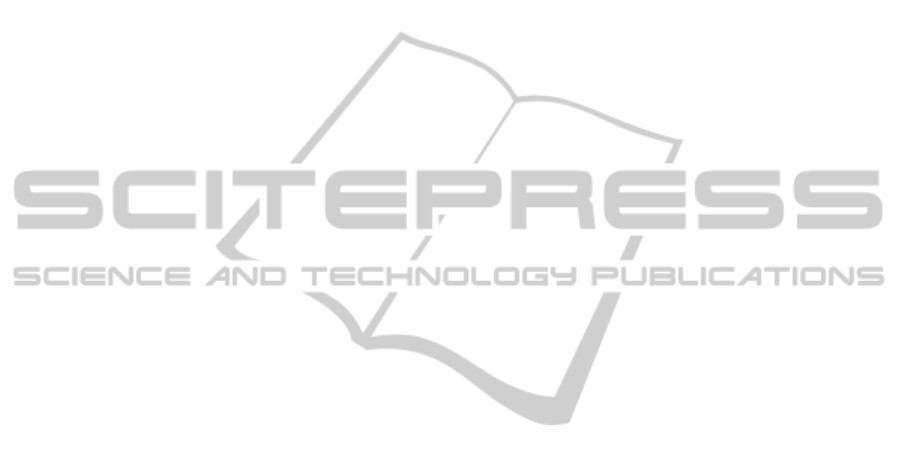
its bases in a central effect of FES that responds to
differences in the lesion and consequent recovery at
the central nervous system level. Further studies are
required to investigate the relationship between the
carryover effect and what is happening in terms of
plasticity and/or connectivity between the involved
areas at central nervous system level.
This work is preliminary and in particular two
further issues would be of interest. Firstly the
treatment only lasts 20 sessions, and it has been
proposed that the longer the treatment the better the
results (Schuhfried et al., 2012). It could therefore be
interesting to follow up the evolutions of the
carryover effect along a longitudinal study.
Moreover, a validation of the carryover effect
quantitative definition by a group of clinicians that
separately assess the presence/absence of carryover
would be an interesting further development.
It is interesting to note that this quantitative
method could be applied to any other group of
outcome measures in order to define the carryover
effect on any other particular district (e.g. upper
limbs)
5 CONCLUSIONS
The proposed method allows to quantitatively
distinguish patients that report a carryover effect
following an FES-based treatment for FD. The two
groups are easily identified thanks to clear
mathematical steps based on principal component
analysis that starts from a battery of outcome
measures. In our group of post-stroke chronic
patients, 50% reported a carryover effect after 20
sessions of FES-based treatment. This could inform
further studies aimed at identifying the carryover
effect mechanism of action.
ACKNOWLEDGEMENTS
This work was made possible thanks to the patients
that volunteered to participate to the project, thanks
to Mauro Casarin and Stefano Tagliaferri that gave
their availability to help with scanning.
REFERENCES
Abdi, H., Williams, L.J., 2010. Principal component
analysis. Wiley Interdisciplinary Reviews:
Computational Statistics 2: 433–459.
Ambrosini, E., Ferrante, S., Pedrocchi, A., Ferrigno, G.,
Molteni, F., 2011. Cycling Induced by Electrical
Stimulation Improves Motor Recovery in Postacute
Hemiparetic Patients: a Randomized Controlled Trial.
Stroke 42:1068–1073.
Ashworth, B., 1964. Preliminary trial of carisoprodol in
multiple sclerosis. The Practitioner 192: 540–542.
Burridge, J. H., Taylor, P. N., Hagan, S. A., Swain, I. D.,
1997. The effects of common peroneal stimulation on
the effort and speed of walking: a randomized
controlled trial with chronic hemiplegic patients. Clin
Rehabil. 11:201–210.
Burridge, J. H., Wood, D. E., Taylor, P. N., McLellan D.
L., 2001. Indices to describe different muscle
activation patterns, identified during treadmill
walking, in people with spastic drop-foot. Medical
Engineering and Physics 23: 427–434.
Davis, R. B., Ounpuu, S., Tyburski, D. J., Gage J. R.,
1991. A gait analysis data collection and reduction
technique. Hum. Mov. Sci. 10: 575-587.
Eng, J. J., Dawson, A. S., Chu, K. S., 2004. Submaximal
exercise in persons with stroke: test-retest reliability
and concurrent validity with maximal oxygen
consumption. Arch Phys Med Rehabil 85: 113-118.
Everaert, D. G., Thompson, A. K., Chong, S. L., Stein, R.
B., 2010. Does functional electrical stimulation for
foot drop strengthen corticospinal connections?
Neurorehabil Neural Repair. 24: 168-77.
Fulk, G. D, Echternach, J. L., 2008. Test-retest reliability
and minimal detectable change of gait speed in
individuals undergoing rehabilitation after stroke. J
Neurol Phys Ther 32: 8-13.
Ilgin, D., Ozalevli, S., Kilinc, O., Sevinc, C., Cimrin, A.
H., Ucan, E.S., 2011. Gait speed as a functional
capacity indicator in patients with chronic obstructive
pulmonary disease. Annals of Thoracic Medicine 6:
141-146.
Kesar, T. M., Perumal, R., Jancosko, A., Reisman, D. S.,
Rudolph, K. S., Higginson, J. S., Binder-Macleod, S.
A., 2010. Novel Patterns of Functional Electrical
Stimulation Have an Immediate Effect on Dorsiflexor
Muscle Function During Gait for People Poststroke.
Physical Therapy 90: 55-66.
Kesar, T. M., Binder-Macleod, S. A., Hicks, G. E.,
Reisman, D. S., 2011. Minimal detectable change for
gait variables collected during treadmill walking in
individuals post-stroke. Gait Posture 33: 314-317.
Kottink, A. I., Oostendorp, L. J., Buurke, J. H., Nene,
A.V., Hermens, H.J., IJzerman, M.J., 2004. The
orthotic effect of functional electrical stimulation on
the improvement of walking in stroke patients with a
dropped foot: a systematic review. Artif Organs
28:577–586.
Liberson, W. T., Holmquest, H. J., Scot, D., Dow, M.,
1961. Functional electrotherapy: stimulation of the
peroneal nerve synchronized with the swing phase of
the gait of hemiplegic patients. Arch Phys Med
Rehabil. 42:101–105.
Lin, P., Yang, Y., Cheng, S., Wang, R., 2006. The
Relation Between Ankle Impairments and Gait
IJCCI2013-InternationalJointConferenceonComputationalIntelligence
566