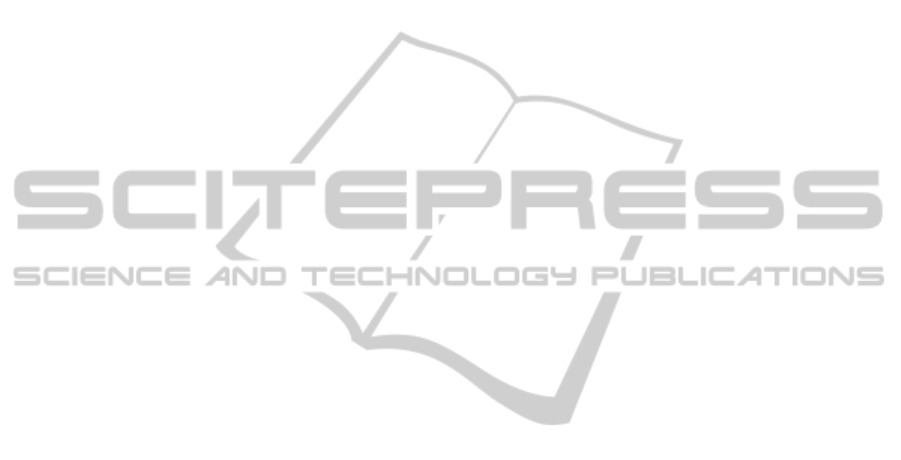
Goldenberg, G. and Hagmann, S. (1997) The meaning of
meaningless gestures: a study of visuo-imitative
apraxia. Neuropsychologia, 35(3): 333–341.
Goldenberg, G., & Hagmann, S. (1998) Therapy of
activities of daily living in patients with apraxia.
Neuropsychological Rehabilitation, 8(2), 123–41.
Goldenberg, G., Hermsdörfer, J., & Spatt, J. (1996).
Ideomotor apraxia and cerebral dominance for motor
control. Cognitive Brain Research, 3, 95-100.
Graessel, E., Viegas, R., Stemmer, R., Küchly, B.,
Kornhuber, J. & Donath, C., (2009). The Erlangen
Test of Activities of Daily Living: first results on
reliability and validity of a short performance test to
measure fundamental activities of daily living in
dementia patients. International Psychogeriatrics, 21,
103-112.
Haaland, K.Y., Harrington, D.L., (1994). Limb-
sequencing deficits after left but not right hemisphere
damage. Brain and Cognition, 24, 104-22.
Hanna-Pladdy, B., Heilman, K.M., & Foundas, A.L.
(2003) Ecological implications of ideomotor apraxia:
evidence from physical activities of daily living.
Neurology, 60, 487–490.
Hermsdörfer, J., Mai, N., Spatt, J., Marquardt, C.,
Veltkamp, R., & Goldenberg, G. (1996) Kinematic
analysis of movement imitation in apraxia, Brain, 119,
1575-1586.
Hermsdörfer, J., Ullrich, S., Marquardt, C., Goldenberg,
G., & Mai, N. (1999). Prehension with the ipsilesional
hand after unilateral brain damage. Cortex, 35, 139-
161.
Hermsdörfer, J., Blankenfeld, H., & Goldenberg, G.
(2003). The dependence of ipsilesional aiming deficits
on task demands, lesioned hemisphere, and apraxia.
Neuropsychologia, 41, 1628-1643.
Hermsdörfer, J., Hentze, S., & Goldenberg, G. (2006)
Spatial and kinematic features of apraxic movement
depend on the mode of execution. Neuropsychologia,
44, 1642–1652.
Hermsdörfer, J., Li, Y., Randerath, J., Roby-Brami, A., &
Goldenberg, G. (2012). Tool use kinematics across
different modes of execution. Implications for action
representation and apraxia. Cortex, in press.
Hermsdörfer, J., Bieńkiewicz, M., Cogollor, J., Russel,
M., Baptiste, E., Parekh, M., Wing, A., Ferre, M.,
Hughes, C., (2013). CogWatch - Automated
Assistance and Rehabilitation of Stroke-Induced
Action Disorders in the Home Environment. HCI (17):
343-350 .
Hughes, C., Baber, C., Bieńkiewicz, M., Hermsdörfer, J.
(2013). Application of Human Error Identification
(HEI) Techniques to Cognitive Rehabilitation in
Stroke Patients with Limb Apraxia. HCI (8) , 463-471.
Jason, G. W. (1983). Hemispheric asymmetries in motor
function: Left hemisphere specialization for memory
but not performance. Neuropsychologia, 21 (1), 35-45.
Laimgruber, K., Goldenberg, G., & Hermsdörfer, J. (2005)
Manual and hemispheric asymmetries in the execution
of actual and pantomimed prehension,
Neuropsychologia, 43(5), 682-692.
MATLAB and Statistics Toolbox Release 2012b, The
MathWorks, Inc., Natick, Massachusetts, United
States.
Petreska, B., Adriani, M., Blanke, O. & Billard,
A. (2007) Apraxia: a review. In C. von Hofsten (Ed.).
From Action to Cognition. Progress in Brain Research.
Elsevier. Amsterdam. Vol. 164, pp. 61-83.
Poizner, H., Clark, M.A., Merians, A.S., Macauley, B.,
Rothi, L.J.G. and Heilman, K.M. (1995) Joint
,coordination deficits in limb apraxia. Brain, 118: 227–
242.
Schwartz, M. F., Montgomery, M. W., Fitzpatrick-
desalme, E. J., Ochipa, C., Coslett, H. B., & Mayer, N.
H. (1995). Analysis of a disorder of everyday action.
Cognitive Neuropsychology, 12, 863-892.
Taubin, G. (1991). Estimation Of Planar Curves, Surfaces
And Nonplanar Space Curves Defined By Implicit
Equations, With Applications To Edge And Range
Image Segmentation. IEEE Trans. PAMI, 13, 1115-
1138.
Tretriluxana, J., Gordon, J., Fisher, B. E., & Winstein, C.
J. (2009). Hemisphere specific impairments in reach-
to-grasp control after stroke: effects of object size.
Neurorehabilitation and Neural Repair, 23, 679-691.
Vanbellingen, T., Kersten, B., Van de Winckel, A.,
Bellion, M., et al. (2011). A new bedside test of
gestures in stroke: the apraxia screen of TULIA
(AST). Journal of Neurology, Neurosurgery and
Psychiatry 82(4), 389-92.
BIOSIGNALS2014-InternationalConferenceonBio-inspiredSystemsandSignalProcessing
300