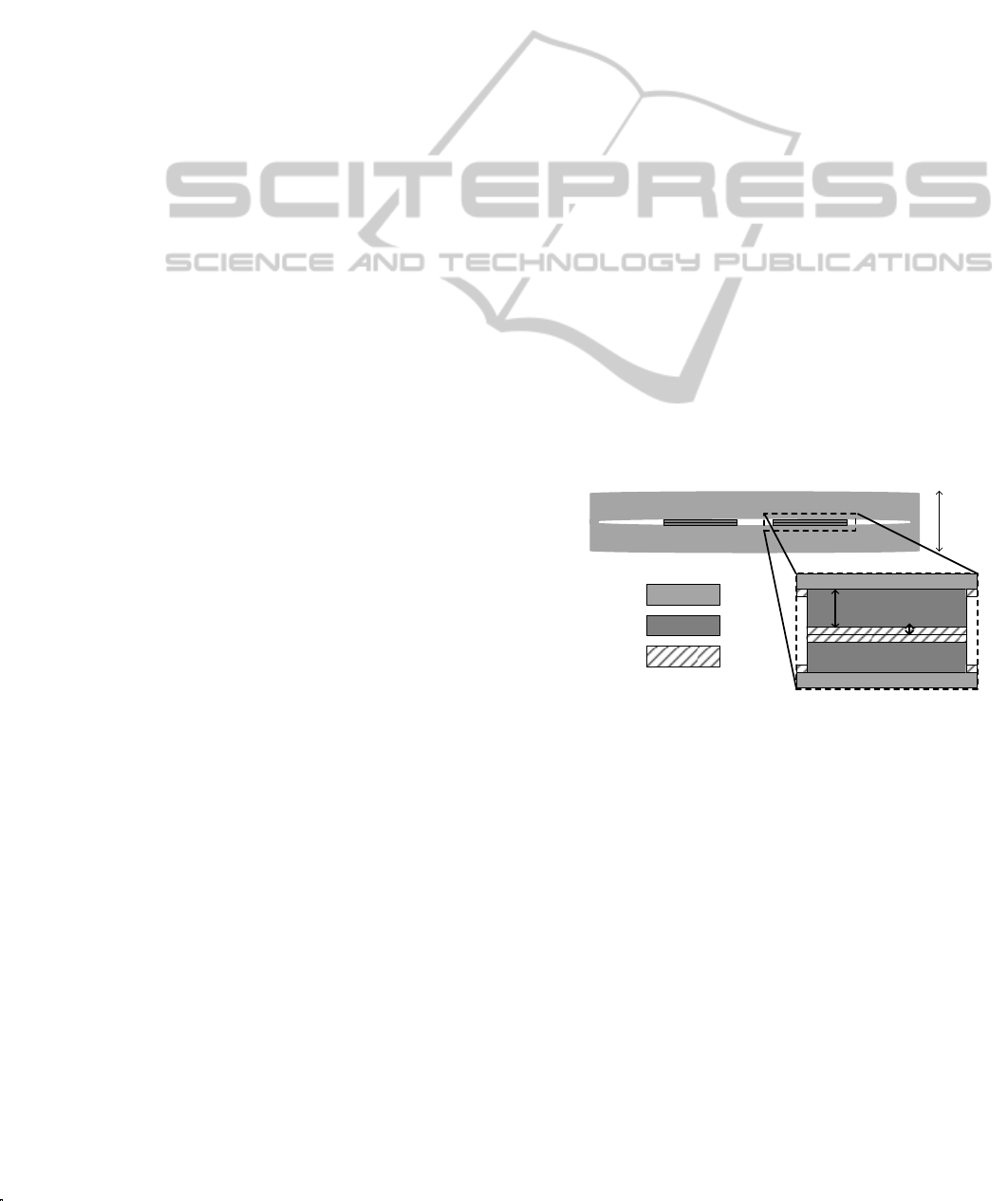
capacitors (Hwang et al. 2012).
The possible substrate materials for biodegra-
dable sensors can be polymers, silk and
bioresorbable glass. Biodegradable polymers are
divided into two groups: synthetic and those derived
from natural resources. There are also two
dominating degradation mechanisms: surface
erosion or bulk erosion. The surface eroding
polymers would make excellent candidates for
sensor fabrication because their water absorption
may be lower. One of the commonly used and well
tested group of biodegradable polymer polylactides,
(PLAs), unfortunately, degrades with bulk erosion.
Other suitable candidates for biodegradable polymer
substrates are polycaprolactone (PCL),
polyglycolide PGA, poly(3-hydroxybutyrate) (PHB)
and their co-polymers (Luo et al. 2014). Luo et al.
used Poly (L-lactide) PLLA as substrate material for
the passive resonance sensors. Another tested
substrate material for biodegradable sensors is
solution-casted silk (Hwang et al. 2012). They also
proposed the use of MgO
and SiO
2
as isolating
materials which are needed to coat conductors.
Besides individual fabrication processes, an
engineering problem arises with the assembly of the
fabricated structures. In comparison to a typical
silicon sensor fabrication, biodegradable structures
are prone to get compromised when new processing
steps are done over earlier layers. As an example,
magnesium layers break if any following steps
include water and many polymer structures will
deform if they get in contact with solvents. To solve
this problem, evaporation processes using physical
masks can be utilized (Hwang et al. 2012). This
method does not include photoresist masks which
have to be patterned and then removed by solvent.
Another method is to use embossing and lamination
techniques (Luo et al. 2014).
In this paper, ongoing research for methods of
designing and fabricating biodegradable passive
resonance sensors is discussed. The methods of
biodegradable sensor fabrication are developed and
combined in a novel way to create a biodegradable
resonator structure that can be modified to make
implantable sensors. The designed prototype
structure is assembled and tested in vitro.
2 FABRICATION METHODS AND
DESIGN
The principal idea of the presented biodegradable
resonator concept is to evaporate magnesium and
silicon dioxide on PLA sheets through a physical
mask. Then two sheets are stacked and combined to
a resonator structure by heat sealing. This method
encapsulates magnesium layers which are otherwise
prone to water. Another leading design feature is to
avoid galvanic through hole vias between the
conductive layers. Instead, conductive layers on the
separate sheets are connected by capacitors which
are formed when the sheets are heat sealed together.
The simplified cross-section of the design is shown
in Fig 1.
The electrical schematic diagram of the designed
circuit is presented in the Fig. 2a. This structure has
four coil and two capacitor structures. The schematic
does not include parasitic components. The
measured electrical behaviour of the presented
structure is similar to a simple RLC circuit and thus
can be roughly modelled as shown in Fig. 2b. The
resistance (Rs) is representing both the ohmic losses
in the magnesium layers and the dielectric losses
around and in the circuit. The inductor in the model
(Ls) represents the combination of four circular
planar coils (L1 to L4) on two opposing PLA sheets
and the inductance of the other connecting wires in
the circuit. In the final structure, four coils are
placed in a way that a changing uniform magnetic
field will induce currents that charge two main
capacitances in the circuit. The capacitor (Cs) in the
model is representing the capacitance of the two
electrode areas that are located inside the circular
coils and the combination of all parasitic
capacitances in the structure. The Fig. 2b also
illustrates the utilized wireless measurement
principle.
Figure 1: The cross-section of the resonator structure.
The idea behind this design can be derived from
a concept where a planar coil and a parallel-plate
capacitor are combined to an LC circuit by using a
through hole via between the layers. Instead of via,
another similar capacitor can be used to connect
layers. The capacitors are in series which has to be
taken account when the resonance characteristics are
designed. This kind of design, however, would be
unsymmetrical and in practice it would take almost
similar area as two planar coils and two parallel-
PLA
Mg
SiO2
50 nm
7.5 μ m
1 mm
BIODEVICES2015-InternationalConferenceonBiomedicalElectronicsandDevices
128