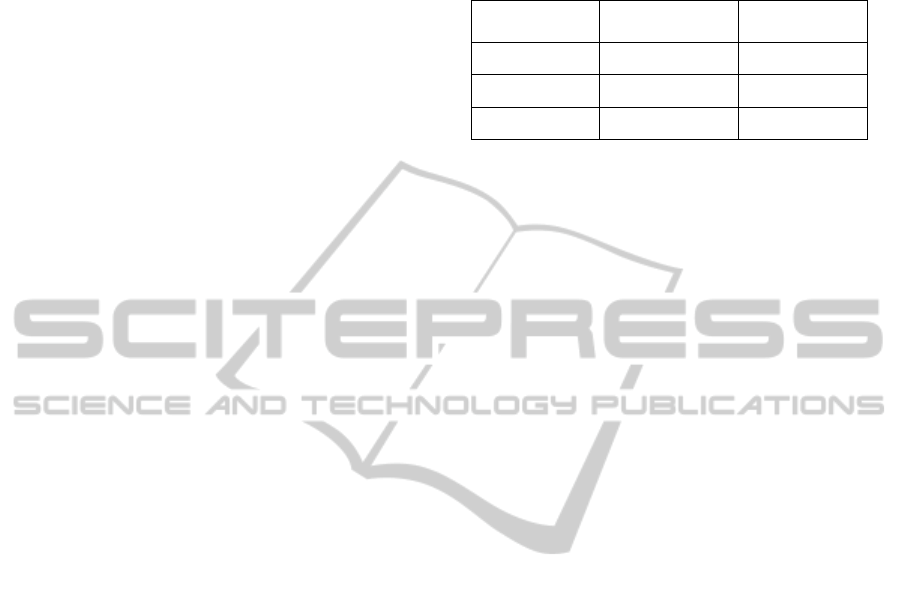
over the three dimensions (x, y, z) from the 10-bits
analog to digital converter. The verification code is
in this case obtained by summation of the first five
MSB of accelerometer data over the three axes,
patient identifier and measure increment. This
scheme leads to T
p
≈ 20 ms. In addition, data are
transmitted each 0,1s so that T
p
/ T
e
is around 20%.
The forward current in this case is fixed to 150 mA,
and the average emitted power is less than 4 mW.
3 EXPERIMENTAL RESULTS
To evaluate the wireless optical technology
performance, we have measured packet loss during
mobile transmission within the room presented in
figure 1, for the two scenarios previously described.
We have performed several measurements with
different persons equipped with the two kinds of
devices and different conditions of lighting from sun
and neon lights at room ceiling. Moreover, during
the test, persons moved regularly throughout the
environment.
A packet can be lost for two reasons: the signal is
not received or it is bad received. The signal is not
received if its amplitude is too low to be detected by
the photo-detector, for example when an obstacle
blocks the optical beam. A packet is bad received
when it contains an error so that the receiver rejects
it. An error is detected as soon as the redundant
patient identifier or the verification code is wrong.
Performance is presented in terms of packet loss
rate determined by the number of lost packets over
the total number of packets sent.
We have evaluated the transmission performance
for one active receiver, two and four. The obtained
results are reported on table I, averaged from 10
measurements for each scenario. The measurements
were carried out over a period of 30 min in order to
detect at least 10 packet losses which are necessary
to have reliable paquet loss rate information. For one
active receiver, measures have been performed for
each receiver A to D. For the case with two receivers
we have considered the couples A-C and B-D
corresponding to room diagonals.
As expected, we can see that the performance
improves when the number of receivers increases.
When there are only one or two receivers,
scenario 2 leads significantly to more packet losses
than scenario 1. This can be explained by the fact
that due to the higher ratio T
p
/ T
e
in scenario 2, the
forward current in the photodiode is lower than in
scenario 1, which means a lower peak optical
emitted power. In addition, the emitter position is
not the same on the patient body so that the body can
be more blocking in scenario 2.
Table 1: Packet Loss Rate.
Number of
receivers
Scenario 1 Scenario 2
4 1,3.10
-3
1,3.10
-3
2 4.10
-3
1,5.10
-2
1 4.10
-2
0,11
When the number of receivers increases, we first
remark for scenario 2 that the performance is
significantly enhanced. Indeed, the packet loss rate
has been improved by one decade by comparing the
results obtained from 1 to 2 receivers and also from
2 to 4. On the other hand, we can note that for
scenario 1 this improvement is not so important: the
packet loss rate from 2 to 4 receivers is only divided
by 3. In addition it can be noticed that with 4
receivers, performance between both scenarios tends
towards a similar value. These observations can be
explained by the fact that, in scenario 1, as the peak
optical emitted power is larger, the optimal room
coverage is achieved with fewer receivers than in
scenario 2.
Finally, our experimental results show that it is
important to take into account the data packet
parameters in order to deploy the right number of
receivers allowing a given quality of service in terms
of packet loss while optimizing the emitted power.
Besides, we can say that the value obtained with
4 receivers, which is 10
-3
, is a typical one in wireless
transmission system and permits concluding that the
wireless optical transmission reliability is ensured.
4 CONCLUSIONS
In this paper, we have presented the experimental
performance of an alternative solution to radio
frequencies for healthcare mobile monitoring that is
wireless optical technology. We have used as a
criterion the packet loss of the transmitted data for
two health-related monitoring scenarios having
different constraints.
For both scenarios, we have investigated the
wireless data transmission between a mobile emitter
worn by the patient and receivers fixed in the
environment, by exploiting optical diffuse
reflections over the room surfaces.
The measurement results obtained with two
prototypes made of commercially available and low
cost components have shown that the optical
HEALTHINF2015-InternationalConferenceonHealthInformatics
556