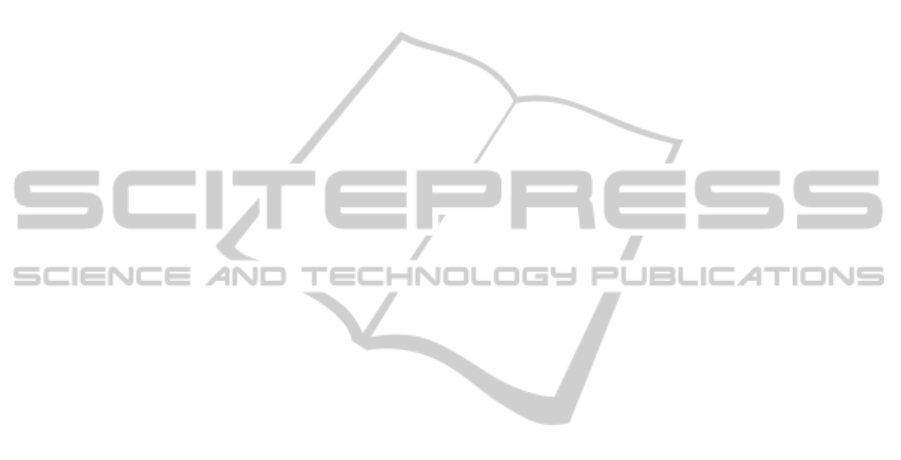
REFERENCES
Abreu, D. C., Cliquet Jr. A., Rondina, J. M., Cendes, F.,
2009. Electrical stimulation during gait promotes
increase of muscle cross-sectional area in
quadriplegics.
Clinical Orthopaedics and Related
Research,
467, pp. 553-557.
Accelerometer ACH-04-98-05, 1998. Measurement
Specialties: Datasheet. Available in: <
http://www.meas-spec.com/>. Accessed in: March,
2008.
Accelerometer, 1999. Piezo Film Sensors - Technical
Manual. Norristown, PA: Measurement Specialties,
Inc..
ACH-04-08-05 (Multi-Axis) and ACH-04-08-05 Analog
Test PCB. Piezo Film Sensors - Product Guide and
Price List. Norristown, PA: Measurement Specialties,
Inc., 2001.
Badj, T., Vodovnik, L., 1984. Pendulum testing of
spasticity
. Journal of Biomedical Engineering, v.6, pp.
9-16.
Carvalho, D. C., Martins, C. L., Cardoso, S. D., Cliquet
Jr., A., 2006. Improvement of metabolic and
cardiorespiratory responses through treadmill gait
training with neuromuscular electrical stimulation in
quadriplegic subjects.
Artificial Organs, 30, pp. 56-63.
Chuang, L., Wu, C., Lin, K., 2012. Myotonometric
Measurement of Muscular Properties of Hemiparetic
Arms ins Stroke Patients. In: Chong-Tae Kim,
Rehabilitation Medicine, InTech. Rijeka, 1
st
edition.
Dietz, V., 2008. Body weight supported gait training: from
laboratory to clinical setting.
Brain Research Bulletin.
v. 74, pp. 459-463.
Esteves, A. C., Stolt, L. R. O. G., Leite, R. M., Dos Reis,
D. C., Borges Jr, N. G., 2007. Validação de um
eletrogoniômetro Digital. Available in: <
http://www2.rc.unesp.br/eventos/educacao_fisica/bio
mecanica2007/upload/115-10-A-
ELETROGONIOMETRO%20IDENTIFICADO.pdf>.
Accessed in: July, 2012.
Guyton, A. C. M. D, 1997.
Tratado de fisiologia médica,
Guanabara Koogan. Rio de Janeiro, 9
th
edition.
Kirshblum, S. C., Burns, S. P., Biering-Sorensen, F.,
Donovan, W., Graves, D. E., Jha, A., Johansen, M.,
Jones, L., Krassioukov, A., Mulcahey, M. J., Read, M.
S., Waring, W., 2011. International standards for
neurological classification of spinal cord injury.
The
Journal of Spinal Cord Medicine,
v. 34, pp. 535-546.
Leitão, A. V., Musse, C. A. I., Granero, L. H. M.,
Rossetto, R., Pavan, K., Lianza, S., 2006.
Espasticidade: Avaliação Clínica.
Sociedade
Brasileira de Medicina Física e Reabilitação - Projeto
Diretrizes.
Lianza, S., Pavan, K., Lourenço, A. F., Fonseca, A.P.,
Leitão, A. V., Musse, C. A. I., Santos, C. A., Masiero,
D., Quagliato, E., Fonseca Filho, G. A., Granero, L. H.
M., Gianni, M. A. C., Gal, P. L. M., Rosetto, R.,
Belizzi, D., Greve, J. M. D, Sposito, M. M. M., 2001.
Diagnóstico e Tratamento da Espasticidade.
Sociedade
Brasileira de Medicina Física e Reabilitação - Projeto
Diretrizes.
Liu, T., Inoue, Y., Shibata, K., Zheng, R., 2010. Wearable
Sensor System for Human Dynamics Analysis. In:
Annalisa Milella, Donato di Paola, Grazia Cicirelli,
Mechatronic Systems Applications, InTech. Vukovar,
1
st
edition.
Miyazaki, E. G., Rosa, T. da S., Nascimento, A. P. H.,
Oberg, T. D., 2008. Influência da estimulação elétrica
funcional para adequação de tono muscular e controle
motor em hemiplégicos.
INTELLECTUS – Revista
Acadêmica Digital do Grupo POLIS Educacional,
Ano 04, n.05, pp. 2-3.
S700 Joint Angle SHAPE SENSOR. S700/S720 Joint
Angle SHAPE SENSOR - Instruction Manual.
Fredericton, NB: Measurand Inc., 2001.
Salmela, L. F. T., Nadeau, S., Olney, S. J., Brouwer, B,
2002. Pêndulo: um teste simples de medida de
espasticidade.
Acta Fisiátrica, v.9, pp. 63-70.
Stillman, B., McMeeken, J., 1995. A video-based version
of the pendulum test: technique and normal response.
Archives of physical medicine and rehabilitation, v.76,
pp. 166-176.
Teive, H. A. G., Zonta, M., Kumagai, Y., 1998.
Tratamento da espasticidade: uma atualização.
Arquivos de Neuro-Psiquiatria, v.56, n.4, pp. 852-858.
Yakamoto, Y., Jikuya, K., Kusuhara Toshimasa.,
Nakamura, T., Michinishi, H., Okamoto, T., 2012.
Precise Measurement System for Knee Joint Motion
During the Pendulum Test Using Two Linear
Accelerometers. In: Md. Zahurul Haq,
Advanced
Topics in Measurement
, InTech. Rijeka, 1
st
edition.
BIODEVICES2013-InternationalConferenceonBiomedicalElectronicsandDevices
126