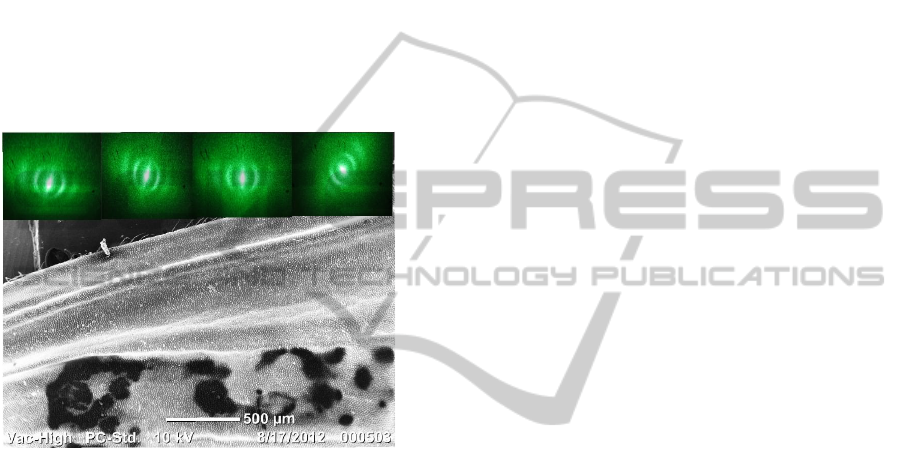
The diffraction pattern on various length scales
reveal how the higher order peaks and their
distribution reflect the structural symmetry and
correlation between these hooks. This disorder
arrangement will results in broadening of diffraction
pattern. As illustrated in Fig 3, the diffraction by a
single hook is compared with the corresponding
diffraction from an array of hooks at various length
scales on the wing surface.
Furthermore, we have observed the rotation of
the diffraction pattern when one translates the beam
spot across the wing as shown in Fig. 4. This
unusual behavior reflects both the local and global
patterns in the variation of the distribution
symmetry.
Figure 4: SEM image of the wing, the inset on the top of
the image are the rotated diffraction pattern profile at
various regions on the wing surface.
It is possible that by varying the spot size from few
microns to several mm, one can obtain the spatial
correlation in a high throughput, single-shot manner.
If such information is attempted by SEM imaging it
would be very tedious and inefficient process. The
spatial correlation of the light and high sensitivity of
the diffraction pattern offer unique advantage over
other methods. It is therefore a potentially attractive
optical technique to unravel the natural design of the
photonic architecture of the insect wing and probe
their functional relationship that ultimately dictates
the motive of symmetry and correlation.
4 CONCLUSIONS
In summary, the proposed optical technique may
prove to be a powerful alternative to gain a better
understanding about the systematic of photonic
architecture such as long range spatial correlations
and symmetry in the insect wings. Our finding
directly demonstrates how the transmitted diffraction
pattern from the wing is correlated with the internal
structural symmetry. The rotations of diffraction
pattern were obtained when the laser beam scanned
various regions on the wing surface as shown in Fig.
4. These rotations of the pattern give the signature of
the spatial symmetry in structural arrangements in
the local to global length scale. These optical tools
could be crucial to understand design principles of
natural photonic crystals with potential applications
for mimicking artificial structures (Mathias et al.,
2010); (Bar-cohen, 2011) that may lead to novel
optical devices
ACKNOWLEDGEMENTS
All authors thank to the DST and IISER Mohali,
India for supporting this research through grant and
research fellowship for Dr. Pramod Kumar. The
invaluable help of Babita Basoya and Gopal Verma
are grateful acknowledged.
REFERENCES
Paris, Andrew D., Chery ,U., Y., Morris, William G.,
Vert, A., Zalyubovskiy, S., Deng, T., Ghiradella,
Helen T. and Potyrailo, Radislav A., 2012. Towards
high-speed imaging of infrared photons with bio-
inspired nanoarchitectures. Nature Photonics 6, 195–
200.
Mika, F., Matějková-Plšková, J., Suratwadee Jiwajinda,
S., Dechkrong, P., Shiojiri, M., Photonic Crystal
Structure and Coloration of Wing Scales of Butterflies
Exhibiting Selective Wavelength Iridescenc.,2012.
Materials 5, 754-771.
Parker, R., A., Natural photonics for industrial
inspiration.,2009. Phil. Trans. R. Soc. A 367, 1759-
1782.
Xu, Q., Po Dong, P., Lipson, M., Breaking the delay-
bandwidth limit in a photonic structure. 2007, nature
Physics 3, 406 – 410.
Wiederhecker, S., Long Chen, Alexander Gondarenko &
Michal Lipson, 2009. Controlling photonic structures
using optical forces. Nature 462, 633-636.
Mathias Kolle, M., Salgard-Cunha, P., M., Scherer, M.,
R., J., Huang, F., Vukusic, P., Mahajan, S., J.
Baumberg, J., Steiner, U., 2010. Mimicking the
colourful wing scale structure of the Papilio blumei
butterfly. nature nanotech. 5 , 511-515.
Shevtsovaa, E., Hanssona, C., Janzenc, H., G.,
Kjærandsend, J., 2011. Stable structural color
patterns displayed on transparent insect wings. Proc
Natl. Acad. Sci. USA. 108(2), 668-673.
UnveilingSpatialCorrelationsinBiophotonicArchitectureofTransparentInsectWings
109