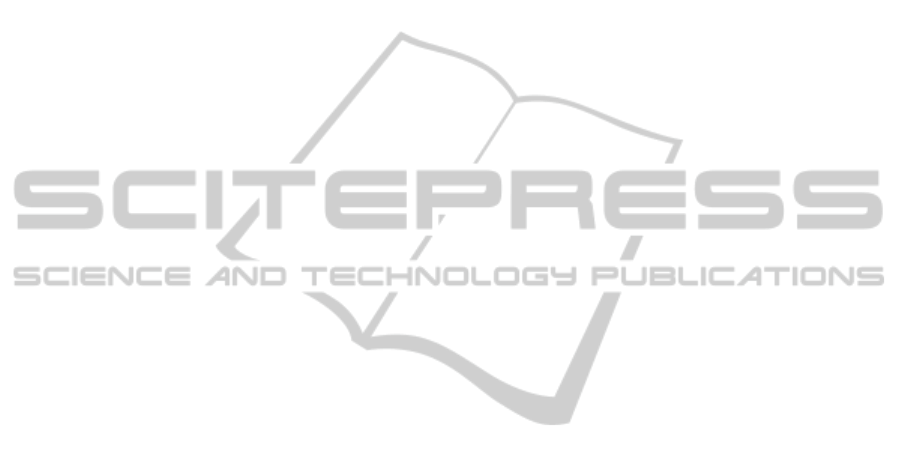
discipline by itself (McNally, 2013). However, one
major challenge asscoiated with the growth of this
discipline is the substantial cost required to provide
laboratory experiences. These hands-on practices are
essential to experience nanoscale matter (atoms and
molecules), and to design nanodevices and systems.
The associated cost can be reduced significantly by
relying on reusable electronic simulations and Web-
based repository of concept resources.
Another typical aspect in nanotechnology educa-
tion, which is not well supported in existing digital
instructional approaches, is the “zoom” effect, which
hierarchically and gradually reveals the infinitesimal
structures of nanomaterial. An alternative “assembly”
effect could empower existing digital instruction to
get learners exposed to both bottom-up and top-down
approaches of nanostructures manufacturing. In this
paper, we propose to augment existing learning tech-
nology standards for supportingthe design and the de-
velopment of virtual learning environments.
We adopt standard structures to organize digital
instructional contents and processes, such as IEEE
LOM (Atif et al., 2003) and IMS SCORM (Hsu et al.,
2010). In doing so, we aim at streamlining the prop-
agation of reusable repositories across the open Web
to facilitate the integration of nanotechnology learn-
ing resources into the rising social trend of Massively
Open Online Courses (or MOOCs) (Zhang, 2013).
2 BACKGROUND AND RELATED
WORKS
There are several challenges facing the integration of
nanotechnology into the mainstream of undergradu-
ate engineering curriculum. First, it is important to
increase the awareness of high school and the first
year engineering students about how the nanotech-
nology will shape our future. To this extend, Jones
et al. (Jones et al., 2003) investigated the feasibility
of allowing students in high school classrooms to con-
duct nanotechnology experiments through controlling
remotely scientific equipments over the Web. Stu-
dents had access to the nanoManipulator tool which
gave them the ability to control an atomic force mi-
croscope over the Internet. The authors believe that
most students were excited about the experience and
developed more accurate concepts regarding nanome-
ter scale as well as 2D and 3D virus morphology.
Another study funded by NSF focused on in-
creasing the nanotechnology awareness for both high
school and first year engineering students (Rodgers
et al., 2013)showed that student had difficulties
defining nanotechnology and its scale. This study
also highlighted computer graphics, visualized siz-
ing charts, and educational videos as effective tech-
niques for helping students understand nanotechnol-
ogy. Moreover,the study showed that connectingnan-
otechnology to various science and engineering fields
could serve as a catalyst method for introducing and
increasing students’ awareness and understanding of
nanotechnology scope.
To reduce the anticipated lab cost, (Sarangan et al.,
2013) suggested the use of a computer based nanofab
trainer. The proposed trainer would allow students
to practice real-life processes and tools as opposed to
normal simulators used for predicting physical phe-
nomena. They also proposed a multimedia system
to bring live interactive demonstrations from exist-
ing nanotechnology laboratories and cleanrooms to
the classrooms. Molecular Workbench software is an-
other tool proposed by Xie and Lee (Xie and Lee,
2012) for teaching nanotechnology concepts. The
tool provides a virtual laboratory in which simulated
nanoscale processes can be examined and manipu-
lated on a computer screen in real time. The au-
thors conducted a pilot study iwhich showed that
simulation-based experimentations can be success-
fully used for undergraduate students to develop an
integrated understanding of concepts in nanotechnol-
ogy at their own pace.
At the same time there are some efforts to incorpo-
rate nanotechnology into the mainstream of an under-
graduate engineering curriculum. Uddin and Chowd-
hury (Uddin and Chowdhury, 2001) proposed the con-
tent of three fundamental courses to be integrated into
an undergraduate engineering curriculum and sug-
gested that the concepts of nanotechnology should be
introduced during freshman and sophomore engineer-
ing courses. They also suggested modifying the out-
comes of junior and senior design courses to include
the modeling, simulation, control and optimization of
nanodevices and systems.
However, the common factor in the above instruc-
tional design approaches is the lack of a standard plat-
form for Web based education to create a space for
educators to share experiences and to reach a wide
community of learners.
Electronic learning production is multilayered as
shown in Figure 1. The core layer is the learning
object, which subsumes learning items following a
standard vocabulary defined by IEEE LOM specifi-
cation. Each item, in turn, points to a resource in
the resources layer. As illustrated in Figure 1, some
resources may reference files or contents outside the
packaged contents through a URL.
Learning objects metadata is a standard structure
to describe educational objects. The IEEE LOM stan-
NanoscaleEducationforSemiconductorDesign
521