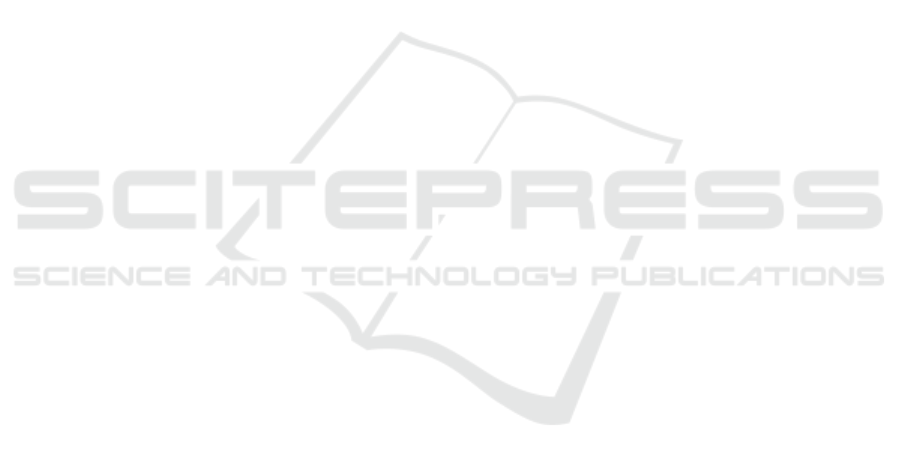
communications in FSO scenario, able to secure tra-
ditional Internet traffic. We demonstrated, by setting
a typical scenario, which limits can be reached by the
considered FSO technology.
REFERENCES
Adhikari, T., Ghosh, A., Khan, A. K., Laha, S., Mitra,
P., and Karmakar, R. (2021). Quantum resistance
for cryptographic keys in classical cryptosystems: A
study on qkd protocols. In 2021 12th International
Conference on Computing Communication and Net-
working Technologies (ICCCNT), pages 1–7. IEEE.
Ai, X., Malaney, R., and Ng, S. X. (2020). A reconcilia-
tion strategy for real-time satellite-based qkd. IEEE
Communications Letters, 24(5):1062–1066.
Barua, B., Hossain, M. M., Islam, M. R., and Bashar, M. K.
(2011). Performance evaluation of different type of
channel models in fso communication. International
Journal of Science and Advanced Technology, 1(5).
Basudewa, M., Bagaskara, Z., Damita, S., Putra, R., and
Ahmadi, D. (2020). Bit error rate performance analy-
sis for free space optic communication. In IOP Con-
ference Series: Materials Science and Engineering,
volume 850, page 012056. IOP Publishing.
Campanile, L., Gribaudo, M., Iacono, M., Marulli, F., and
Mastroianni, M. (2020). Computer network simula-
tion with ns-3: A systematic literature review. Elec-
tronics, 9:272.
Chen, L., Zhang, Z., Zhao, M., Yu, K., and Liu, S. (2022).
Apr-qkdn: A quantum key distribution network rout-
ing scheme based on application priority ranking. En-
tropy, 24(11).
De Rango, F., Tropea, M., Fazio, P., and Marano, S. (2008).
Call admission control for aggregate mpeg-2 traffic
over multimedia geo-satellite networks. IEEE Trans-
actions on Broadcasting, 54(3):612–622.
De Rango, F., Veltri, F., and Fazio, P. (2012). A multipath
fading channel model for underwater shallow acoustic
communications. In 2012 IEEE International Con-
ference on Communications (ICC), pages 3811–3815.
IEEE.
Elser, D., Gunthner, K., Khan, I., Stiller, B., Marquardt, C.,
Leuchs, G., Saucke, K., Trondle, D., Heine, F., Seel,
S., et al. (2015). Satellite quantum communication via
the alphasat laser communication terminal-quantum
signals from 36 thousand kilometers above earth. In
2015 IEEE international conference on space optical
systems and applications (ICSOS), pages 1–4. IEEE.
Epple, B. (2010). Simplified channel model for simulation
of free-space optical communications. Journal of Op-
tical Communications and networking, 2(5):293–304.
Esmail, M. A., Fathallah, H., and Alouini, M.-S. (2016).
Channel modeling and performance evaluation of fso
communication systems in fog. In 2016 23rd In-
ternational Conference on Telecommunications (ICT),
pages 1–5. IEEE.
Fazio, P., Tropea, M., Sottile, C., and Lupia, A. (2015).
Vehicular networking and channel modeling: a new
markovian approach. In 2015 12th Annual IEEE Con-
sumer Communications and Networking Conference
(CCNC), pages 702–707. IEEE.
Ghassemlooy, Z., Popoola, W., and Rajbhandari, S. (2019).
Optical wireless communications: system and channel
modelling with Matlab®. CRC press.
Guo, W., Zhan, Y., Tsiftsis, T. A., and Yang, L. (2022).
Performance and channel modeling optimization for
hovering uav-assisted fso links. Journal of Lightwave
Technology, 40(15):4999–5012.
Ivanov, H., Hatab, Z., and Leitgeb, E. (2021). Channel
emulation of satellite-to-ground apd-based fso link in
the presence of atmospheric turbulence fading. In
2021 16th International Conference on Telecommuni-
cations (ConTEL), pages 33–36. IEEE.
Ivanov, H., Marzano, F., Leitgeb, E., and Bekhrad, P.
(2022). Testbed emulator of satellite-to-ground fso
downlink affected by atmospheric seeing including
scintillations and clouds. Electronics, 11(7):1102.
Jarangal, E. and Dhawan, D. (2018). Comparison of chan-
nel models based on atmospheric turbulences of fso
system-a review. International Journal of Research
in Electronics and Computer Engineering, 6(1):282–
286.
Jia, Z., Zhu, Q., and Ao, F. (2006). Atmospheric attenu-
ation analysis in the fso link. In 2006 international
conference on communication technology, pages 1–4.
IEEE.
Kamal, M., Khan, J., Khan, Y., Ali, F., Armghan, A.,
Muhammad, F., Ullah, N., and Alotaibi, S. (2022).
Free space optics transmission performance enhance-
ment for sustaining 5g high capacity data services. Mi-
cromachines, 13(8):1248.
Kaushal, H., Jain, V., Kar, S., Kaushal, H., Jain, V., and
Kar, S. (2017). Free-space optical channel models.
Free space optical communication, pages 41–89.
Kupferman, J. and Arnon, S. (2018). Zero-error attacks on a
quantum key distribution fso system. OSA Continuum,
1(3):1079–1086.
Mehic, M., Fazio, P., Rass, S., Maurhart, O., Peev, M.,
Poppe, A., Rozhon, J., Niemiec, M., and Voznak, M.
(2019). A novel approach to quality-of-service pro-
visioning in trusted relay quantum key distribution
networks. IEEE/ACM Transactions on Networking,
28(1):168–181.
Mehic, M., Niemiec, M., Rass, S., Ma, J., Peev, M.,
Aguado, A., Martin, V., Schauer, S., Poppe, A.,
Pacher, C., and Voznak, M. (2020). Quantum key dis-
tribution: A networking perspective. ACM Comput.
Surv., 53(5).
Moosavi, S. A. and Saghafifar, H. (2018). Irradiance fluctu-
ations of an indoor fso channel based on hot air cham-
bers. Optik, 173:110–119.
Najafi, M., Ajam, H., Jamali, V., Diamantoulakis, P. D.,
Karagiannidis, G. K., and Schober, R. (2020). Sta-
tistical modeling of the fso fronthaul channel for uav-
based communications. IEEE Transactions on Com-
munications, 68(6):3720–3736.
Performance Evaluation of Free Space Optics Laser Communications for 5G and Beyond Secure Network Connections
257