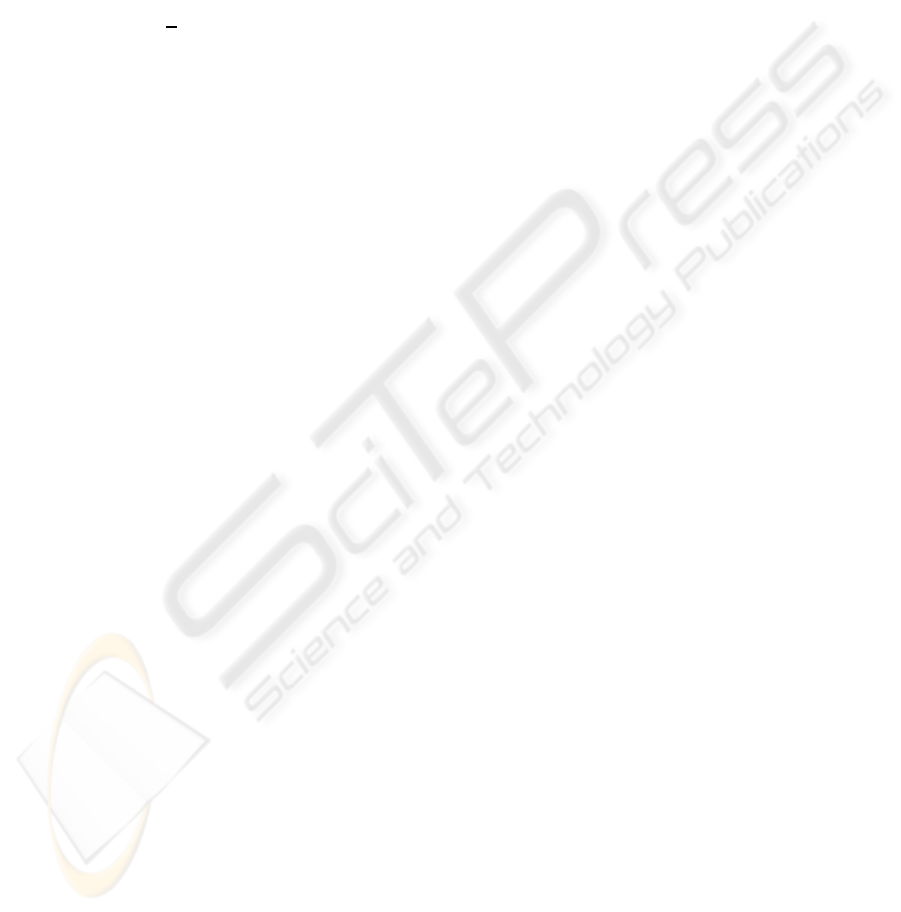
is noticeable that the cortical acceleration frequency
spectrum is higher than the others.
4.2 Energy
From the acceleration signal recorded, the kinetic en-
ergy of the Ti6Al4V disc/accelerometer unit during
the mechanical shock is computed with the classical
formula
E
k
=
1
2
m
Z
v(t)
2
dt.
(with E
k
: kinetic energy, m: mass , v: speed, given for
each disc-accelerometer unit.)
The speeds of the different disc-accelerometer
units are computed by integration of the acceleration
signals, which occurs in the data processing sequence
after application of 30 Hz Butterworth high-pass fil-
ter to remove continuous component. The frequency
response curve of the Butterworth filter is practically
flat in the passband. However, the use of this kind
of filter introduces a non linear frequency dephasing.
In this study, only the frequency content is expected
and not the signal phase, thus the application of But-
terworth filter is well adapted. The kinetic energy is
calculated per surface unit: Ti6Al4V, 4.8±0.1 pJ/µm
2
for 1 Hps and 50.2±1.1 pJ/µm
2
for 10 Hps; HAP,
2.0±0.04 pJ/µm
2
for 1 Hps and 20.6±0.6 pJ/µm
2
for
10 Hps; Cortical bone, 8.5±0.2 pJ/µm
2
for 1 Hps
and 65.1±3.6 pJ/µm
2
for 10 Hps; Trabecular bone,
0.9±0.2 pJ/µm
2
for 1 Hps and 8.8±3.2 pJ/µm
2
for 10
Hps. Notice that values of kinetic energy at 10 Hps
are 10 times higher than those at 1 Hps.
5 SYNTHESIS
This new BSD was initially developed to simulate the
effects of mechanical impacts on bone cells cultured
on biomaterials to compare them with the effects of
impacts during walking or running activities. A pre-
vious study on Ground Reaction Forces (GRF) (Gi-
akas G, 2001) has shown that frequency content dur-
ing impact phase of running is limited to 100 Hz, ac-
celeration magnitude is ∼10 g. During shocks ap-
plied with the BSD, a frequency range comprised be-
tween 100 Hz and 4 kHz, an acceleration magnitude
of 783,1± 32,5 g at 1 Hps and an acceleration time of
∼1 ms have been found on Ti6Al4V for example. So
it is quite difficult to directly put in relation the results
obtained during this kind of mechanical impact with
those obtained by GRF studies.
In this study, recording conditions for acceleration
and force signals were slightly different from condi-
tions used in case of in vitro experiments, particularly
concerning the sealing of the culture chamber.
The disc should be excited by a frequency higher
than his resonnant frequency to enter in resonnance.
To verify this assumption, a numerical simulation was
perfomed and the first resonant frequency mode of
the Ti6Al4V disc was found to be at 144 kHz, which
is much higher than the frequencies observed on the
force signal Fourier transform. Consequently, the disc
can not enter in resonance and induces some self-
frequencies. The results found during signal record-
ing can be extrapolated to in vitro conditions.
When mechanical shocks were applied to the
discs, whatever the signal stimulation frequency was
(1 Hps and 10 Hps), one could observe that the shape
and the mean maximal value of the force and accel-
eration signals were identical. Analysis of accelera-
tion signals leads to an estimation of the kinetic en-
ergy of the tested disc during one impact. It has been
found that the amount of kinetic energy at 10 Hps is
10 times greater than that calculated at 1 Hps. Consid-
ering these results, in the case of mechanical shock,
it appears that it is more interesting to analyse more
accurately the frequency content of force signals, to
compare kinetic energy derived from accelerations
signals, and to focus on the force perceived by cells
when they are subjected to acceleration phase. By ap-
plying the fundamental law of dynamic, the acceler-
ation force during impact has been calculated by es-
timating cellular mass (1 picogramm): a force of ∼1
nN per cell has been found. This acceleration force
is comparable to forces for which biological events
have been previously observed. Consequently, it can
be expected that this new kind of mechanical stimula-
tion would have biological effects on bone cells.
A critical analysis can be done concerning the
reproducibility of the acceleration and force peaks’
measurements. The values and their errors are for
each material: Ti6Al4V, 783±31 g; HAP, 1197±87 g;
Cortical bone, 1215±21 g; Trabecular bone, 225±24
g; and for each force signals: Ti6Al4V, 34±0.6 N;
HAP, 28±0.2 N; Cortical bone, 23±0.2 N; Trabecu-
lar bone, 8±0.6 N. The error is less important in the
case of hard materials (cortical bone acceleration er-
ror is < 2% for example). However, a 10% error oc-
curs on the measurements of acceleration and force
peaks on the trabecular bone. This can be due to the
non-heterogeneity of the material and probably to the
non-flatness of the surface. In fact, the guidance of
the hammer is not perfect so the head of the hammer
does not hit in the same place every time. This is an
improvement to ensure to the BSD for future work.
An other improvement has been already done, it con-
sists in a screw that allows to ajust the stroke of the
A NEW INSTRUMENTED BIOLOGICAL DEVICE DESIGNED TO APPLY MECHANICAL SHOCKS TO BONE
CELLS
277