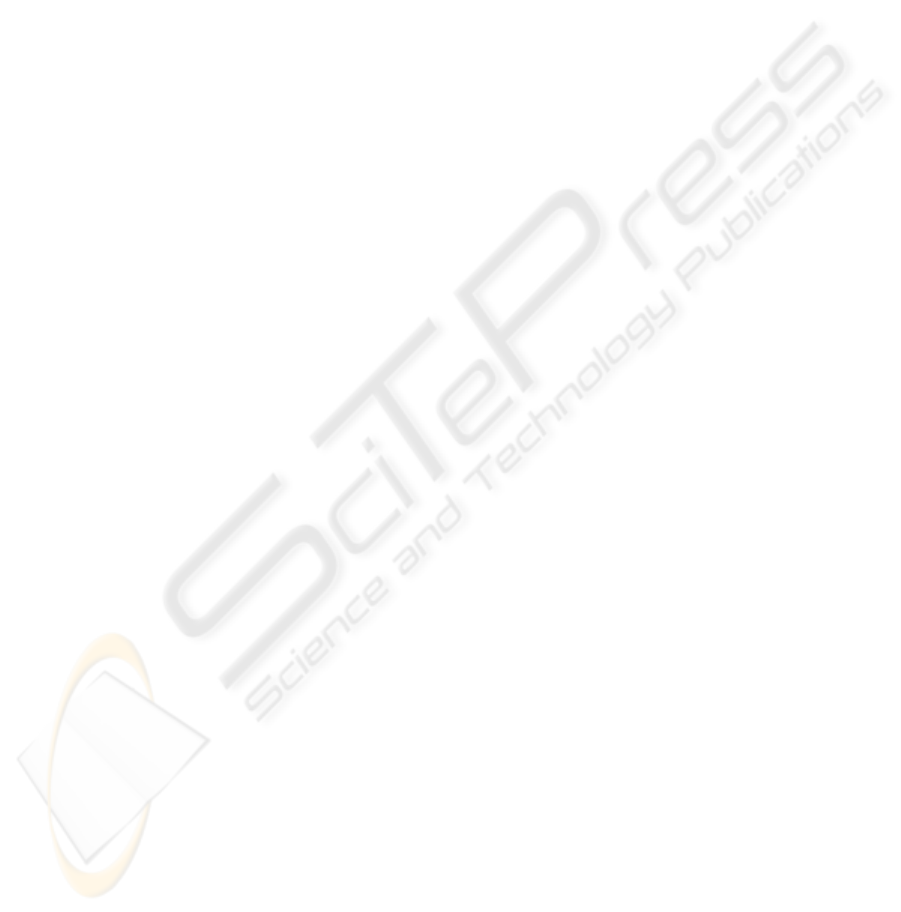
Claycomb J.R. et al., 2002. “Nonlinear Dielectric
Spectroscopy of Living Cell Suspensions”. 2nd joint
EMBS/BMES Conference, Houston, USA.
De Langhe P. et al., 1994. “Design Rules for an
Experimental Setup Using an Open-Ended Coaxial
Probe Based on Theoretical Modelling”. IEEE Trans,
Instrum. Meas., 43, 6.
Duncan L. et al., 2006. “Assessment of the dielectric
properties of drug sensitive and resistant leukaemic
cells before and after ion channel blockers using
dielectrophoresis”. NSTI-Nanotech 2006.
Ermolina I. et al., 2001. “Study of Normal and Malignant
White Blood Cells by Time Domain Dielectric
Spectroscopy”. IEEE Trans. Dielectrics and Electrical
Insulation, 8, 2.
Fan S. et al., 1990. “Static analysis of an Open-Ended
Coaxial Line Terminated by Layered Media”. IEEE
Trans, on Instrum. Meas., 39, 2.
Fear E.C., 2005. “Microwave Imaging of the Breast”.
Tech. Cancer Res. Treatm., 4, 1.
Foster K.R., Schwan H.P., 1996. “Dielectric properties of
tissues” in C. Polk, E. Postow: Handbook of
Biological Effects of Electromagnetic fields, CRC,
Boca Raton, FL.
Gabriel S. et al., 1996. “The dielectric properties of
biological tissues: I. Literature survey - II.
Measurements in the frequency range 10 Hz to
20 GHz - III. Parametric models for the dielectric
spectrum of tissues”. Phys. Med. Biol., 41.
Gascoyne P. R. C. et al., 1997. “Dielectrophoretic
separation of cancer cells from blood”. IEEE Trans. on
industry applic., 33, 3.
Grant J.P. et al., 1989. “A critical study of the open-ended
coaxial line sensor technique for RF and microwave
complex permittivity measurements”. J. Physics E:
Sci. Instrum., 22.
Hagl D.M. et al., 2003. “Sensing Volume of Open-Ended
Coaxial Probes for Dielectric Characterization of
Breast Tissue at Microwave Frequencies”. IEEE
Trans. Microw. Theo. Tech., 51, 4.
Hagness S.C. et al., 1998. “Two-dimensional FDTD
analysis of a pulsed microwave confocal system for
breast cancer detection: Fixed-focus and antenna array
sensors”. IEEE Trans. Biomed. Eng., vol. 28.
Hoshina et al., 2001. “A Numerical Study on the
Measurement Region of an Open-Ended Coaxial
Probe Used for Complex Permittivity Measurement”.
IEEE Trans. Magnetics, 37, 5.
Hübner Y. et al., 2005. “Parallel measurements of drug
actions on Erythrocytes by dielectrophoresis, using a
three-dimensional electrode design”. IEE Proc.
Nanobiotechnol., 152, 4.
Jaspard F. et al., 2003. “Dielectric properties of blood: an
investigation of haematocrit dependence”. Physiol.
Meas., 24.
Joines W.T. et al., 1994. “The measured electrical
properties of normal and malignant human tissues
from 50 to 900 MHz”.
Med. Phys., 21, 4.
Lisin R. et al, 1996. “Time domain dielectric spectroscopy
study of human cells. I. Erythrocytes and ghosts”.
Biochim. Biophys. Acta, vol. 1280.
Pietrucha K., Marzec E., 2005. “Dielectric properties of
the collagen–glycosaminoglycans scaffolds in the
temperature range of thermal decomposition”.
Biophys. Chemistry, 118.
Polevaya Y. et al., 1999. “Time domain dielectric
spectroscopy study of human cells. II. Normal and
malignant white blood cells”. Biochim. Biophys. Acta,
1419.
Santini M.T. et al., 1991. “Effects of lonidamine on the
membrane electrical properties of Ehrlich ascites
tumor cells”. FEBS, 291, 2.
Santini M.T. et al., 1995. “A dielectric Relaxation Study
on the Effects of the Antitumor Drugs Lonidamine and
Rhein on the Membrane Electrical Properties of
Ehrlich Ascites Tumor Cells”. Anticancer Res., 15.
Semenov S.Y. et al., 2000. “Microwave Spectroscopy of
Myocardial Ischemia and Infarction. I. Experimental
Study”. Ann. Biomed. Eng., 28.
Semenov S.Y. et al., 2003. “Microwave Tomography for
Detection/Imaging of Myocardial Infarction. I.
Excised Canine Hearts”. Ann. Biomed. Eng., 31.
Sha L. et al., 2002. “A review of dielectric properties of
normal and malignant breast tissue”. IEEE
SoutheastCon conference, Columbia SC, USA.
Shao W. et al., 2005. “UWB Microwave Imaging for
Breast Tumor Detection in Inhomogeneous Tissue”.
27
th
Conf. IEEE EMBS.
Sheen N.I., Woodhead I.M., 1999. “An Open-ended
Coaxial Probe for Broad-band Permittivity
Measurement of Agricultural Products”. J. Agricult.
Eng. Res., 74.
Smith S.R. et al., 1986. “Dielectric properties of VX-2
Carcinoma Versus Normal Liver Tissue”. IEEE Trans.
Biomed. Eng., 33, 5.
Surowiec A.J. et al., 1988. “Dielectric Properties of Breast
Carcinoma and the Surrounding Tissues”. IEEE Trans.
Biomed. Eng., 35, 4.
Tofighi M.R., Daryoush A.S., 2001. “Near field
microwave brain imaging”. Electron. Lett., 37, 13.
Treo E.F. et al., 2005. “Hematocrit Measurement by
Dielectric Spectroscopy”. IEEE Trans. Biomed. Eng.,
52, 1.
Yang Y. et al, 2004. “Monitoring of lung tumour cell
growth in artificial membranes”. Biosensors and
Bioelectronics, 20.
MICROWAVE DIELECTRIC SPECTROSCOPY OF LOW-VOLUME FRACTION HUMAN CANCER CELLS
EMBEDDED IN COLLAGEN GELS - Experimental Feasibility Study with an Open-ended Coaxial Probe
161