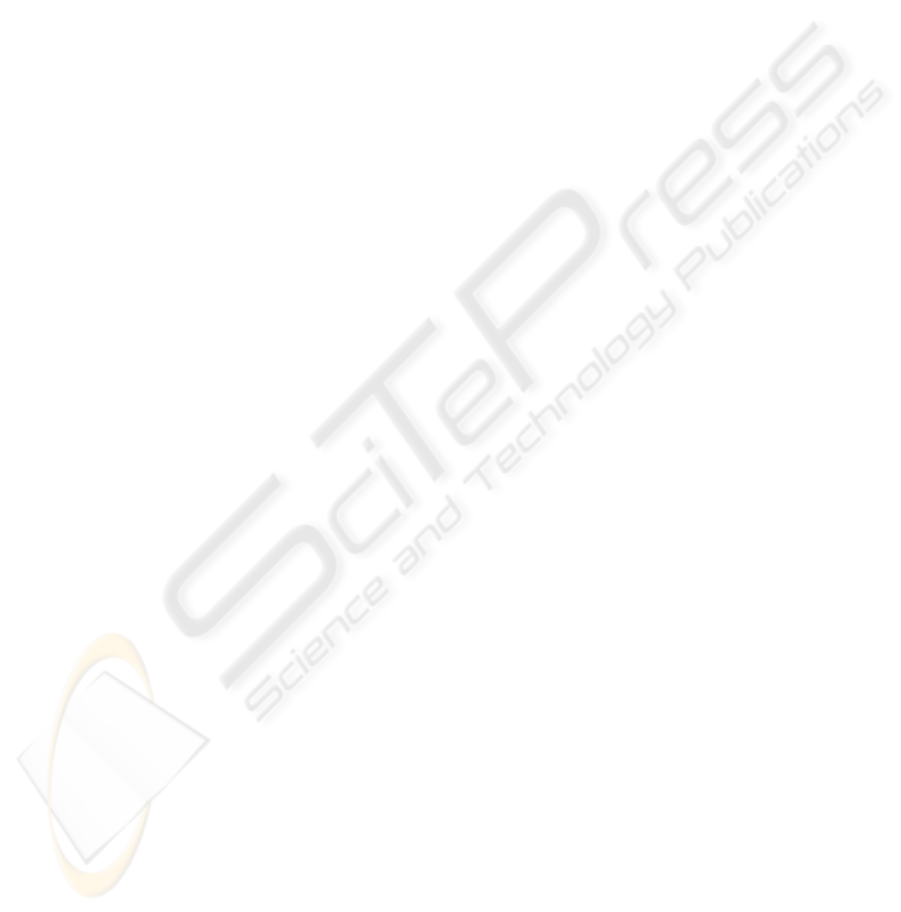
We report on the successful use of surface-FIDA
as diagnostic tool for prion diseases. The infectious
agents of prion diseases are composed primarily of
the pathogenic isoform of the prion protein
designated PrP
Sc
, which is generated by a
conformational change of the cellular isoform PrP
C
.
In contrast to its cellular isoform, the pathogenic
isoform PrP
Sc
forms insoluble aggregates. Hitherto
accredited prion tests use the PK-resistance of PrP
Sc
as a marker for the disease. Because of varying
portions of disease related aggregated PrP, which is
not PK-resistant, these prion tests offer only a
limited sensitivity. Therefore prion protein aggregate
detection, which does not rely on PK-digestion, is
favourable for sensitive diagnosis. It allows
detection of both, PK-resistant and PK-sensitive
PrP
Sc
aggregates.
Up to now, we could successfully verify the
novel test system for correct diagnosis of Scrapie-
infected hamsters as well as BSE-infected cattle in
the clinical stages of diseases (Birkmann et al.,
2007). Furthermore, we were able to detect PrP
aggregates in the cerebrospinal fluid of cattle of
BSE-infected cattle for the first time (Birkmann et
al., 2007). During the next steps we will adopt the
highly sensitive test system for diagnosis of human
prion diseases like Creutzfeldt-Jakob disease and
other aggregate related diseases, especially
Alzheimer’s disease.
In this study we apply surface-FIDA to different
disease associated aggregates. First we show the
single aggregate detection of prion protein
aggregates purified from brain homogenates of
Scrapie-infected hamsters and BSE-infected cattle to
demonstrate the principal of surface-FIDA to detect
single particles. In the second part of the work we
show the transfer of surface-FIDA to the detection
of single Aβ aggregates as diagnostic approach for
Alzheimer’s disease. Therefore we compared the
detection of Aβ aggregates in solution with the
application of surface-FIDA.
2 MATERIALS AND METHODS
2.1 Fluorescence Labelling of
Antibodies
Antibodies R1 were kindly provided by S.B.
Prusiner, UCSF, USA (Williamson et al., 1998).
Antibodies 12F10 and Saf32 were obtained from
SpiBio (Massy Cedex, France); antibody D18 was
obtained from InPro (San Francisco, USA). For the
detection of Aβ aggregates, antibodies 6E10 (Sigma
Aldrich, Hamburg, Germany), 8G7, 19H11 and 4G8
(Calbiochem) were purchased.
Antibodies were labelled in free amino groups
via reactive succinimidyl ester groups of Alexa-633
and Alexa-488 (Molecular Probes, Oregon, USA).
For labelling, approximately 50 µg antibodies were
incubated with 5 µg dye in carbonate buffer, pH 8.4
in a total volume of 100 µl for 1 hour. Conjugates
were separated from free dye by gel filtration via
NAP5-column (Pharmacia) with 10 mM TBS, pH
7.2 and 0.2 M NaCl as elution buffer. Labelled
antibodies were stored in the dark at 4°C.
2.2 Fluorescence Correlation
Spectroscopy
In fluorescence correlation spectroscopy (FCS) the
fluorescence intensity is recorded in a very small
volume, i.e. in the femtoliter range. Measurements
were performed with the instrument FCS Olympus
IX 50 (Evotec OAI, Hamburg, Germany) with a
beam scanner unit in dual-colour mode with an
Argon ion laser (excitation wavelength 488/514 nm)
and a helium-neon laser (excitation wavelength 633
nm). The beam scanner unit allows the scanning of
the sample for aggregates. In practice the detection
volume is moved through the sample in horizontal
and vertical dimensions. The beam scanner was used
by moving 1 mm in one direction a rectangular
deviation of 100 µm with a frequency of 50 Hz and
an integration time of 50 µs. A piezo element was
integrated in the optic of the FCS Olympus IX 50,
which allowed a precise z-positioning of the focus in
the 100 nm range.
2.3 Surface-FIDA
The surface-FIDA assay was carried out as
described by earlier (Birkmann et al., 2007).
Briefly, 0.25 – 1 μg capture antibody was
adhesively bound to poly-D-lysine activated glass
surfaces. After blocking the unspecific binding sites
with 10 % fetal calf serum, potentially present
aggregates were bound to the capture by incubating
20 μl of a sample for at least two hours at 4 °C.
After washing twice with PBS buffer (140 mM
NaCl; 2.7 mM KCl; 10 mM Na
2
HPO
4
, pH 7.4), the
fluorescence labelled detection antibodies were
applied (0.1 μg/μl) and incubated for 1 h at 20 °C.
After five washing steps with PBST (PBS with 0.1
% (w/w) Tween 20) and two washing steps with
PBS, the measurements were carried out.
BIOSIGNALS 2008 - International Conference on Bio-inspired Systems and Signal Processing
432