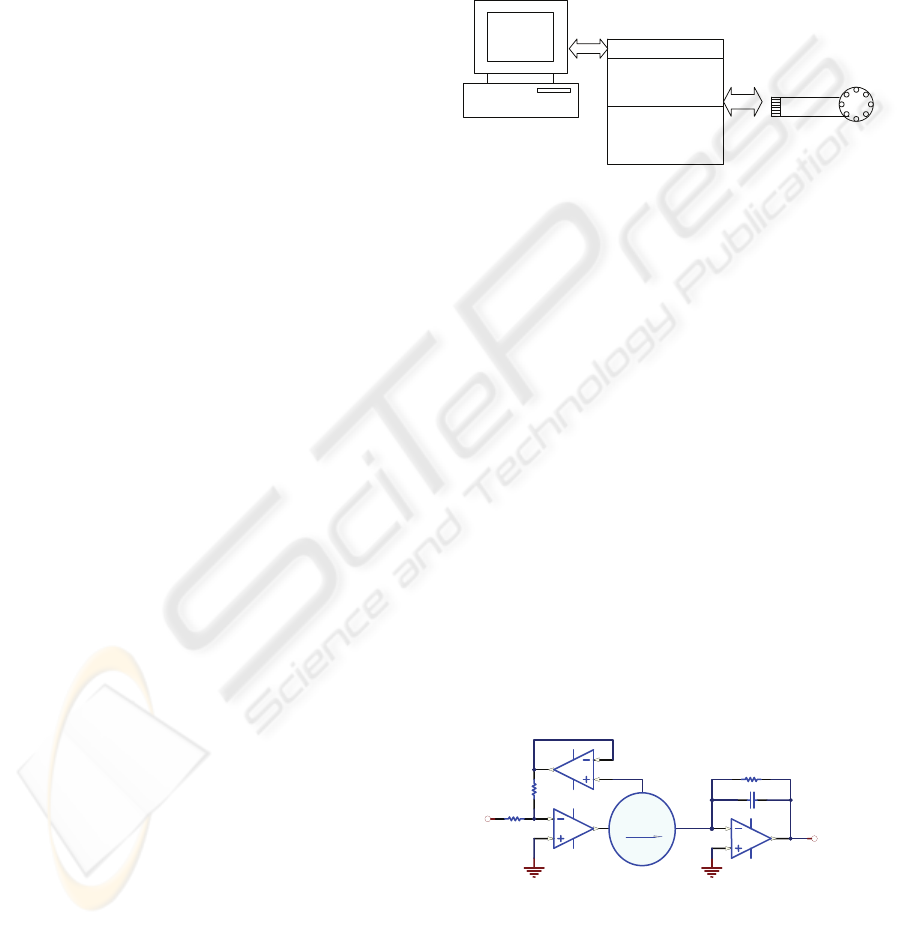
There have been notable advances in suitable
sensors for early stage disease detection (Peng et al.,
2008), (Seo et al., 2008), (Ndamanisha and Guo,
2008), (Wang and Ha, 2007), (McLaughlin et al.,
2002), (Abbaspour and Mirzajani, 2007), (Morales
et al., 2007), (Shiddiky et al., 2008), However, there
remains a need to address the developments in
appropriate systems to allow progression of these
sensors outside of the lab to POC and to in vivo
applications. Currently, sensing instrumentation is
mostly bench-top based equipment, which is large
and not adapted to work outside of the lab
environment. Therefore, the development of
miniaturized sensing instrumentation is essential.
In this study, we discuss approaches to develop
miniaturized sensing systems for in vitro and in vivo
applications based on a potentiostatic solution,
which is commonly used with different
electrochemical sensors, and different sensing
methods (amperometry, voltammetry,
impedometry). Although, fundamentally the systems
for in vitro and in vivo applications are built on
similar circuitry, they vary significantly due to
different requirements in size, power, sensitivity,
biocompatibility and functionality. For the in vitro
applications, we have developed a portable, hand-
held, miniaturized, multichannel potentiostatic
system, which is optimized for operation with a
sensor array on chip. The system has the appropriate
accuracy and sensitivity required by biomedical
applications, and would be suited for use in a
hospital or at a GP’s office. For the in vivo
applications, we have developed two variants of the
system that are both miniaturized and can operate
over extended periods on low power, again with the
required accuracy and sensitivity. The first solution
is based on commercially available low-power, low-
noise, micro-sized small outline integrated circuit
(SOIC) components and chips. The second device
presents a specialized on-chip system that is
developed in-house. The in vivo systems have
similar performance specification but the PCB-based
system costs less. Both of these systems are
optimized for low-power operation, making them
applicable for implantable devices, where an
appliance is implanted and remains long term in the
body, operating on a continued basis.
2 SENSING SYSTEM
STRUCTURE
In general, for both system solutions, the
electrochemical potentiostatic sensing system
consists of an electrochemical cell incorporating the
sensor or sensor array, and two main analog
electronic units, the potentiostat and a
transimpedance amplifier, and a microcontroller
unit. These analog units connect to the
microcontroller unit that controls the measurement
process, and provides data acquisition and
connectivity to the personal computer (PC) as shown
in figure 1.
Microcontroller
Multichannel
potentiostat
Transimpedance
amplifier
Microcell
PC
Figure 1: Block diagram of electrochemical sensing
system for in vitro and in vivo applications.
The electrochemical cell is usually a three
electrode structure comprising a counter electrode
(C), reference electrode (R) and working electrode
(W), which is immersed into or covered by sample
solutions to be analyzed. Electrochemical or
biochemical reactions in the cell are detected with
electronics, in particular with a transimpedance
amplifier. The reactions are dependent on the W
potential, therefore, a stable potential at the W
should be provided. The W potential stability is
secured by the R, which supplies a reference
potential independent of the environment, and the
potentiostatic unit, which maintains this W potential,
with respect to the R, to be equal to a stimulation
signal generated by the microcontroller unit. The
shape of the stimulation signal depends on the
sensing methodologies (i.e. DC for amperometry,
staircase for voltametry and so on). A simplified
schematic of the electrochemical potentiostatic
sensing system (without microcontroller unit) is
shown in figure 2
Figure 2: Simplified schematic of the potentiostatic
sensing system.
Implementation of the electrochemical sensing
systems for in vitro and in vivo biomedical
applications is each governed by a different set of
requirements. For in vitro real- time systems, these
2
3
1
Op3
W
Vout
2
3
1
Op2
2
3
1
Op1 C
Vin
Current pass
CELL
BIODEVICES 2009 - International Conference on Biomedical Electronics and Devices
84