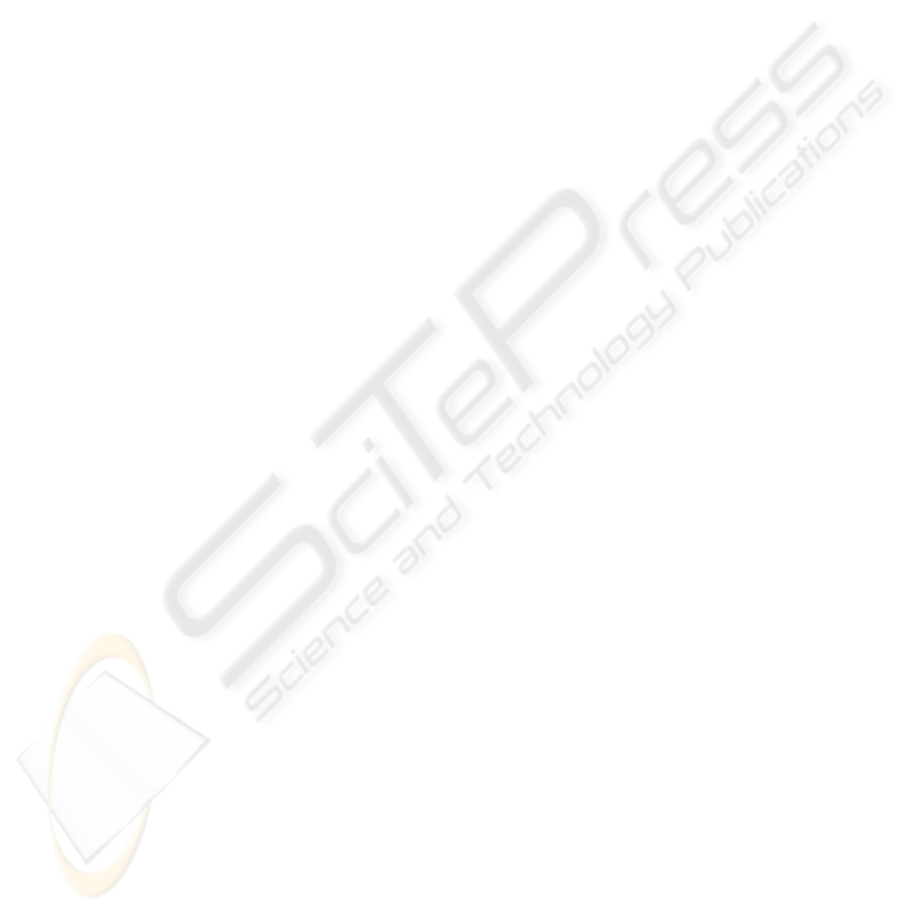
peatable exposition at a fixed distance from the object
and different collimator parameter settings prove that
changes in the physical properties of X-ray beams oc-
cur. Proof of the existence of a dependency between
the volume of ferrofluid in the lens and the lens’ capa-
bility for modifying the parameters of the permeating
X-ray beam was of significant importance in confirm-
ing the influence of ferrofluid on the physical proper-
ties of an X-ray bundle. This may in the future lead
to the creation of lenses for various practical applica-
tions. A non-linear dependency has also been found
between the collimator’s rotational speed and its abil-
ity to modify the parameters of the permeating X-ray
beam. This feature seems to be best evidence that
interference occurs at closely quantized electron or-
bits of atoms belonging to the rotating quasi-crystal
of the ferrofluid as it should be remembered that if
the crystal was understood to operate as a simple stop,
the characteristics would most probably have a linear
character. Therefore the clear dependency between
the modifying properties of the collimator and the ro-
tational speed, exluding changes in the volume of fer-
rofluid, seems to be of significant value for the fu-
ture perspective of creating easily-controllable units,
serially produced devices broadening the diagnostic
capabilities of standard X-ray tubes or enhancing the
further development of work on gradeint tomography.
Although the research results have been quite interest-
ing, it is important to bear in mind that the presented
scientific evidence concerning the modification of an
X-ray bundle has an intermediate character, this due
to the fact that it deals with a secondary evaluation
of effects induced in the tested object following a
conversion of the ray’s energy into the visible light
range. The main reason why such an approach for
conducting this research was chosen is that the med-
ical community has become used to utilising X-ray
diagnostics in such a manner, as well as for its con-
siderably lower costs. Currently work is underway in
the Laboratory of Biotechnology on the next stage of
research which involves making direct measurements
of difraction and interference in the output X-ray bun-
dle after being modified by the collimator.
REFERENCES
Bankier A, Fleichmann D, Aram L, Heimberger K,
Schindler E, Herold C. Bildgebung in der In-
tensivmedizin Techniken, Indikationen, diagnos-
tische Zeichen. In: Bardenheuer H, Hilfiker O,
Larsen R, Radke J. Weiterbildung fr Ansthesisten.
Springer,Berlin, 15-48
Carlson J. D., Electrorheological Fluids, US Patent 4,772,
407
Czerny C. Steiner E., Gstttner W., Baumgartner WD.,
Imhof H. Postoperative radiographic assessment
of the Combi 40 cochlear implant. Am. Journ.of
Roentgenology 1997:169(6):1689
Dyszkiewicz A, Sapota G, Wrbel Z. Standaryzowana
fotometria zdj radiologicznych ukadu kostnego w
tworzeniu komputerowych algorytmw densytome-
trycznych. Konf. TIM 99, Jaszowiec 1999
Dyszkiewicz A, Wrbel Z. The procedure of supervising
treatment of thyroid gland with isotope j 131 and using
2d and 3d analysis of scintigraphical image. ICXOM
Vienna 2001
Dyszkiewicz A. Procedure for monitoring the evolution of
inflammatory and degeneration changes in sacroiliac-
lumbar joints and correcting the rtg-picture density di-
vergence. ICXOM Vienna 2001
Dyszkiewicz A., Kolumna chromatograficzna do sczenia
lub filtracji, patent PL 175577
Folster R. T., Magnetoorheological Fluids, US Patent
5,667,715
Grampp S. Steiner E. Imhof H. Radiological diagnosis of
osteoporosis. Eur J Radiol 1997a:7:2:S11-9
Grampp H. Genant A. Mathur Ph. Lang M. Jergas M.
Takada C. Gler Y. Lu, M. Chavez Comparison of
noninvasive bone mineral measurements in assessing
age-related loss, fracture discrimination, and diagnos-
tic classification. J Bone Miner Res 12 (5) (1997b):
697
Kainberger F. Mittermaier F. Seidl G. Parth E. Weinstabl
R. Imaging of tendons–adaptation’ degeneration’ rup-
ture. Eur J Radiol 1997:25(3):209
Kollmann, K. Turetschek, W. Backfrieder, G. Most-
beck Quantitative analysis with an amplitude-coded
color Doppler imaging system (in-vitro study). World
Congress on Medical Physics and Biomedical Engi-
neering. Nice, France Sept 14-19, 1997
Kramer J. Hofmann S. Plenk H. Schneider W. Engel A.
Imaging of avascular necrosis of bone. Eur Radiol
1997: 7:2:180
Ławniczak A., Milecki A., Ciecze elektro- i mag-
netoreologiczne oraz ich zastosowania w technice,
Wydawnictwo Politechniki Poznaskiej 1999
Majumdar, H. Genant, S. Grampp, D.C. Newitt, V. Truong,
J.C. Lin, A. Mathur Correlation of trabecular bone
structure with age, bone mineral density, and osteo-
porotic status: in vivo studies in the distal radius using
high resolution magnetic resonance imaging. J Bone
Miner Res 12 (1) (1997): 111
Phule P. P. Magnetoorheological Fluid, US Patent
5,985,168
Rand T. Seidl G. Kainberger F. Resch A. Hittmair K.
Schneider B. Gler CC. Imhof H. Impact of spinal de-
generative changes on the evaluation of bone mineral
density with dual x-ray absorptiometry (DXA). Calc.
Tissue 1997: 60:430
Sasaki M., Ishii T., Haji K, Electrorheological Fluid com-
prising lyotropic liquid crystalline polymer, US Patent
5,746,934
COLLIMATION OF X-RAY DIAGNOSTIC BUNDLE BY MEANS OF STEERING FERROFLUID
447