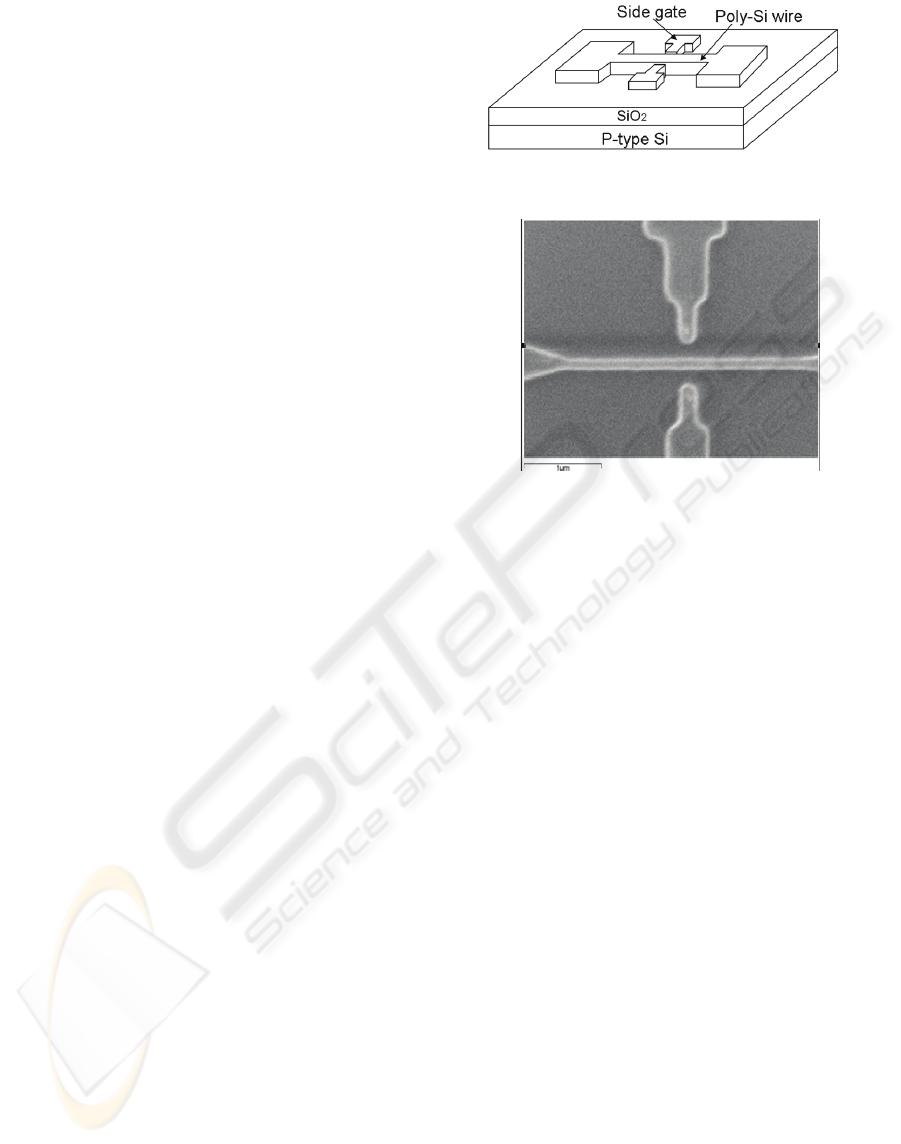
DNAs. Instead of using SiNW, in this work we used
poly-Si wire for sequence-specific ssDNAs as well
as ds DNAs, A, T, C, G, A-T and C-G, detection
from the consideration of simplicity, economy and
easy-fabrication. In the present work, current
flowing through the poly-Si wire channel rather than
time-dependent conductance was determined. We
found that the amount of current change before and
after dropping the DNA solution under test on the
poly-Si wire surface is different for each ssDNA and
dsDNA. This result indicates that each of ssDNAs
and dsDNAs has its own characteristic amount of
current changes, and the poly-Si wire sensor can be
used as a promising DNA detection device.
2 EXPERIMENTS
In this work, p-type (100) Si wafer was used as the
substrate. After standard RCA cleaning, a 12 nm-
thick thermal oxide was grown at 900℃. Following
that, a phosphorous-doped polysilicon layer with a
thickness of 80 nm was deposited at 620℃ by
vertical furnace and having a sheet resistance of 40-
50 Ω/□. An e-beam writer was then used to define
the pattern of the poly-Si wire. After development,
the poly-Si wire was obtained by reactive-ion-
etching. The line width and length of the poly-Si
wire is about 200nm and 2um, respectively. Figure 1
and Figure 2 depicts respectively the schematic
diagram and the SEM image of the poly-Si wire
sensor used in this work. To increase the detection
sensitivity, an enzyme layer (γ-APTES) is deposited
onto the poly-Si wire surface and then cured at
120℃ for 5 min on a hot plate, which can enhance
the adhesion of DNA molecular with the poly-Si
wire surface as well as increase the sensitivity of the
sensor. The same sensor has been reported being
successfully used for cancer cells detection (Wu et al,
2008). In this work, the sensor was used for DNA
detection.
Sequence-specific DNA reagents (Invitrogen,
U.S.A.), ssA, ssT, ssC, ssG, ds(A-T) and ds(C-G),
with different concentrations (50, 100 nM) and
lengths (10, 20 mer) were prepared and dissolved in
a 0.165 M phosphate buffer solution. The DNA
solution under test was then dropped onto the poly-
Si wire sensor surface by using a micropipette. A
voltage was applied between the source and the
drain of the poly-Si wire without side gate bias, and
the current flowing through the poly-Si wire was
measured by using the semiconductor parameter
analyzer HP 4156B. All the experiments were
carried out at room temperature.
Figure 1: Schematic diagram of the poly-Si wire biosensor.
Figure 2: SEM picture of the Poly-Si wire, the line width
and length are about 200nm and 2um, respectively.
3 RESULTS AND DISCUSSION
Figure 3 and Fig. 4 show respectively the I-V
characteristics of 50 nM and 100 nM ssA, ssT, ssC
and ssG, with different lengths under forward bias.
As observed, the current I
DS
flowing through the
poly-Si wire increases with increasing source-drain
voltage V
DS
. It is interesting to note that the current
flowing through the poly-Si wire channel after the
solution under test was dropped onto the poly-Si
wire surface is higher than that before it was
dropped for all the sequence-specific ssDNAs except
for ssC. This result indicates that the polarity of the
surface charge of ssC is opposite to that of other ss
DNAs. Figure 5 compares the absolute value of the
amount of current changes (which is defined as ΔI
=⎥ I
DS
(after dropping) – I
DS
(before dropping)︳) of
ssA, ssT, ssC and ssG DNA with different
concentrations and lengths measured at V
DS
= 5V.
We find that ΔI increases with increasing DNA
length as well as concentration as expected because
the longer length and the higher concentration of
ssDNA, the more charges within the poly-Si wire
channel will be induced. We also observe that, for
the same length and concentration of ssDNA, the
amount of current changes ΔI is that T > C> G > A.
It is reported that the Fermi level of DNA bases is
that T < C < G < A (Zwolak and Di Ventra, 2008).
Therefore, we believe that the surface charge of the
ELECTRICAL CHARACTERIZATION OF SEQUENCE-SPECIFIC LABEL-FREE DNA BY USING POLYSILICON
WIRE
171