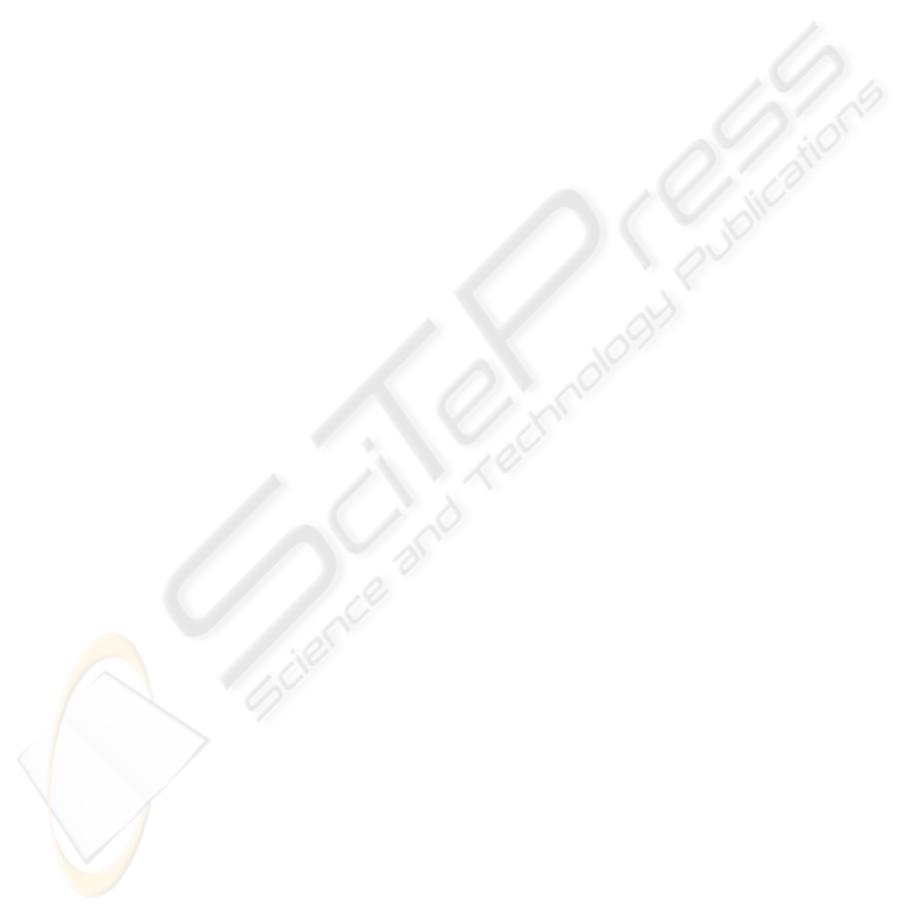
refractive index of the cytoplasm tends to increase
with the degree of maturity. Although the maturity
classes can be visually distinguished, it seems
difficult to use the refractive index value to estimate
the maturity of a completely unknown oocyte. We
proposed a discussion on this issue. We think that,
and this is perhaps an interesting conclusion of this
work, oocytes should not only be qualified in terms
VG, MI and MII but also regarding their temporal
evolution over these maturation stages.
Studies on a much larger number of oocytes are
still required. Also, the investigation of other optical
characteristics should be envisaged. At the end, we
hope that these studies will help biologists in
choosing not only the right oocyte but also the right
oocyte at the right moment.
REFERENCES
Beebe, DJ., Wheeler, M., Zeringue, HC. et al., 2002,
Microfluidic technology for assisted reproduction,
Theriogenology, Vol. 57, pp. 125–135.
Danielli, JF. 1952, Division of the flattened egg, Nature,
Vol. 170, pp. 496-496.
Gauthier, G., Piat, E., 2002, An electromagnetic
micromanipulation system for single cell
manipulation, J. Micromech. Vol. 2, pp. 87–119.
Hwang, H., Lee, DH., Choi, W., et al., 2009, Enhanced
discrimination of normal oocytes using optically
induced pulling-up dielectrophoretic force,
Biomicrofluidics, Vol. 3, pp. 014103-1-014106-10
Inui, H., Nakamura, H., Mizuno, J., et al., 2006,
Development of ovum evaluation system for human
assisted reproductive technology: verification of the
effects and the safety, in 22nd Annual Meeting of the
European Society of Human Reproduction and
Embryology (ESHRE2006), Proceedings in Hum.
Reprod. 1(Suppl 1), pp. i161.
Kricka, LJ., Faro, I., Heyner, S., et al., 1997,
Micromachined analytical devices: microchips for
semen testing, J. Pharm. Biomed. Anal. Vol. 15, pp.
1443–1447.
Marquet, P., Rappaz, B., Magistretti, PJ., et al., 2005,
Digital holographic microscopy: a noninvasive
contrast imaging technique allowing quantitative
visualization of living cells with subwavelength axial
accuracy, Opt. Lett., Vol. 30, pp. 468-470.
Murayama, Y., Omata, S., Constantinou, CE., 2004,
Micro-mechanical sensing platform for the
characterization of the elastic properties of the ovum
via uniaxial measurement, J. Biomech. Vol. 37, pp.
67-72.
Nakamura, S., Hiramoto, Y., 1978, Mechanical properties
of the cell surface in starfish eggs, Dev. Growth
Differ. Vol. 20, pp. 317-327.
Ohtsubo, M., Hiramoto, Y., 1985, Regional differences in
mechanical properties of the cell surface in dividing
echinoderm eggs. Development, Growth and
Differentiation, Dev. Growth Differ. Vol. 27, pp.371-
383.
Oliviera, PL., Kawooya, JK., Ribiero, JMC., et al., 1995,
J. of Biological Chemistry, Vol. 270, pp. 10897-
10901.
Pieralli, C., Wacogne, B., André, C., et al., 2009,
Biological qualification of oocyte maturity with the
use of the Karhunen-Loeve transform: computer aided
decision for selecting best oocytes before fertilization,
in Medical Image Analysis and Description for
Diagnosis Systems, Proceedings of the First
International Workshop on Medical Image Analysis
and Description for Diagnosis Systems, INSTICC
Press, ISBN: 978-989-8111-77-7, pp. 77-84.
Sadani, Z., Wacogne, B., Pieralli, C., et al., 2005,
Microsystems and microfluidic device for single
oocyte transportation and trapping: toward the
automation of in vitro fertilising, Sens. and Act. A
Vol. 121, pp. 364–372.
Sawai, T., Yoneda, M., 1974, Wave of stiffness
propagating along the surface of the newt egg during
cleavage, J. Cell Biol. Vol. 60, pp. 1-7.
Shaked, NT., Rinehart, MT., Wax, A., 2009, Dual
interference channel quantitative phase microscopy of
live cell dynamics, Opt. Lett., Vol. 34, pp. 767-769.
Suh, RS., Phadke, N., Ohl, DA.,
et al., 2003, Rethinking
gamete/embryo isolation and culture with
microfluidics, Human Reproduction Update, Vol. 9,
pp. 451-461.
Wacogne, B., Pieralli, C., Roux, C., et al., 2008,
Measuring the mechanical behaviour of human
oocytes with a very simple SU-8 micro-tool,
Biomedical Microdevices, Vol. 10, pp. 411-419.
Zeggari, R., Wacogne, B., Pieralli, C., et al., 2007, A full
micro-fluidic system for single oocyte manipulation
including an optical sensor for cell maturity estimation
and fertilisation indication, Sens. and Act. B, Vol. 125,
pp. 664-671.
Zeggari, R., Wacogne, B., Pieralli, C., 2006, Optics and
microsystems for in vitro fertilisation, Laser Physics,
Vol. 16, pp. 294-302.
Zeringue, HC., Beebe, DJ., Wheeler, MB., 2001, Removal
of cumulus from mammalian zygotes using
microfluidic techniques, Biomed. Microdev. Vol. 3,
pp. 219–224.
BIODEVICES 2010 - International Conference on Biomedical Electronics and Devices
132