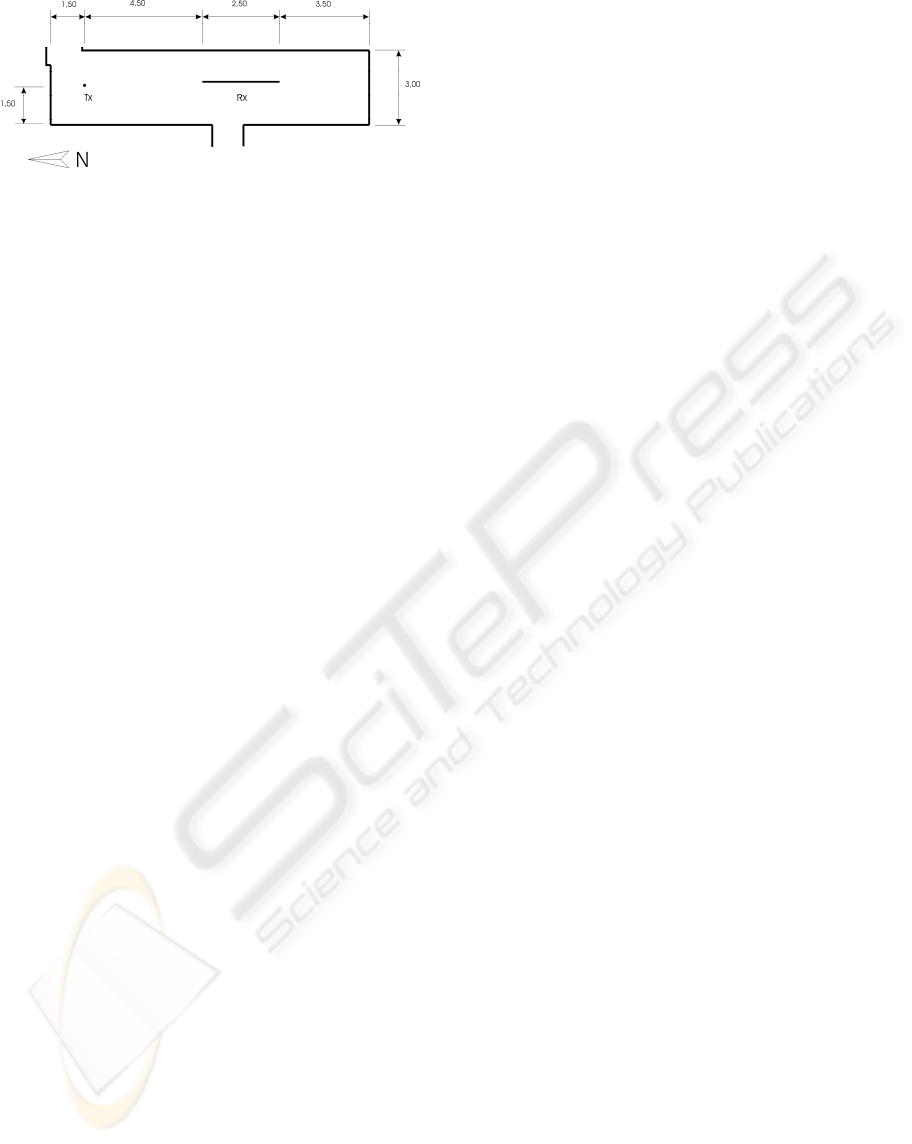
Figure 5: Map of the fourth and fifth measurement
environment.
This environment is more complicated: both North
and South walls are made of brick, the West wall is
made of chip wood in its North half, and brick the
South half, and the East wall is brick constructed,
but it contains a window.
2.3 Measurement Procedure
The transmitting antenna was kept stationary at a
height of 1.8 meter. This location guaranteed that the
radiating element was approximately equidistant
from floor and ceiling.
The receiving antenna was moved along 2.5
meter long linear paths by means of the automatic
positioner. Data were taken every one-eighth of a
wavelength (Dossi et al, 1996), which represent a de
facto standard when measuring radio channels, as
adjacent samples are far enough to be uncorrelated
and they are near enough to keep all fade event.
At each position, complex frequency responses
have been measured in a 160 MHz band around 5.8
GHz, with a resolution of 200 kHz, due to the 801
points in the frequency scan. As a consequence, the
sounder resolution in the delay domain is 6.25 ns,
while the maximum measurable delay is 5 μs.
The measurements were taken following a
procedure “measure-move-stop-measure-...” in order
to avoid Doppler effects within the data.
3 DEPOLARISATION
Indoor environments typically present large
multipath phenomena, which are the main trouble
when planning a wireless network. However, the
depolarisation induced by transmission of waves
across the walls (or by reflection on the walls) is not
commonly taken into account during the planning
procedure. And it could be useful to improve the
performance of the receiving signal if a polarisation
diversity technique is implemented at the reception
end.
This section deals with the fundamentals of
depolarisation and the depolarisation indexes used to
processing the results.
3.1 Depolarisation Phenomenon
A phenomenon associated to reflection, the
depolarisation that could be generated when a wave
beats a flat obstacle, appears to be not so fine
defined and modelled as the reflection itself. This is
probably because typical planning tools, as ray-
tracing, were initially created to be used at
frequencies corresponding to cellular phone or
television broadcasting, at which the typical
obstacles (walls) are electrically flat enough to
provide strong specular reflections.
At higher frequencies, the electrical size of a
given obstacle becomes larger. At 5.8 GHz, as an
example, some simulation tools could not work as
well as expected, because when a wave reaches an
obstacle, several reflection paths are generated in
any directions, not only the specular direction
(Cuiñas et al, 2007). And moreover, the obstacle
depolarises the wave in a certain percentage, which
is not commonly considered in such prediction tools.
3.2 Depolarisation Indexes
The depolarisation index, for any material, at any
angle of incidence and any polarisation of the
transmitted waves is the fraction of the power of this
wave that is received in the orthogonal polarisation.
From this definition, depolarisation indexes may be
computed by means of a matrix procedure (Cuiñas et
al, 2009).
The depolarisation indexes for the reflection
mechanism, computed in the specular direction, are
summarized in table 1. The values depend on the
polarisation of the incident wave, which is denoted
by “h” when it is horizontal and “v” when vertical.
The specular situation is adequate to define very
good reflectors, which reflect most of the incident
wave towards the opposite direction, being the
normal to the surface the axis of symmetry.
The results of table 1 indicate that brick wall
provides reduced depolarised waves compared to the
co-polar reflected waves in the specular direction.
The other considered materials provide depolarised
waves up to 9.8% compared to the co-polar one.
But when a more complex analysis is expected,
as it is the situation of the present paper, all
scattering directions have to be considered, and not
just specular one, because the reflector could be
randomly located and oriented.
WINSYS 2010 - International Conference on Wireless Information Networks and Systems
118