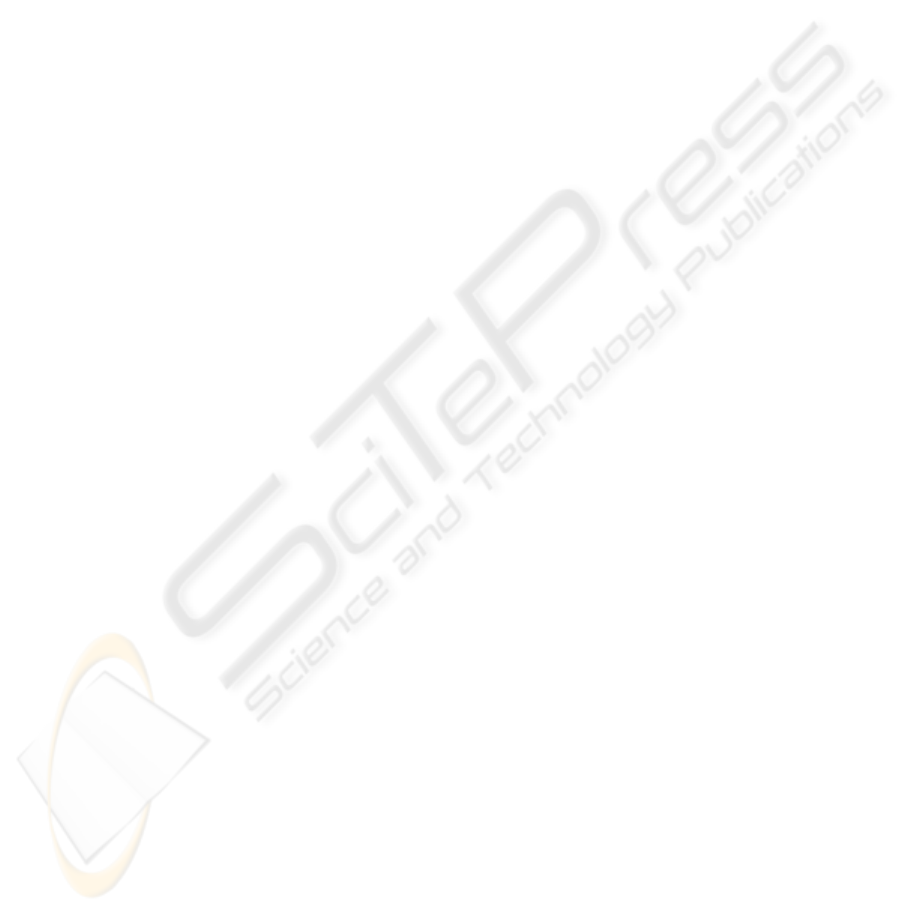
2 ARCHITECTURAL
CONSIDERATIONS
In order to quantify the gains and trade-offs
comparing dynamic solutions vs. static provisioning
we first examine the case of wavelength switching
as a technology to implement the circuit switching
concept (Optical Circuit Switching - OCS). OCS
employs a pre-provisioned allocation of network
resources in order to serve the capacity demand.
OCS inherits both the advantages and disadvantages
of circuit switching: it guarantees QoS with no
losses or delay but at a high overall cost and poor
resource utilisation.
In order to overcome the severe scalability
limitations of OCS we consider sub-wavelength
reservation and switching techniques and evaluate
OBS as an indicative case. OBS, as proposed in the
literature, is a dynamic resource allocation protocol
and statistical multiplexing solution. An OBS node
aggregates traffic destined to one destination and
casts it into a burst after transmitting a reservation
message informing the intermediate nodes for the
upcoming burst transmission. It is well-known
through numerous studies, that OBS cannot
guarantee QoS due to this one-way reservation
scheme that leads to high burst losses. Slotted OBS
(S-OBS), presented by Z. Zhang, L. Liu and
Yuanyuan Yang (2007) is an OBS solution where all
bursts are constrained to a specific size. S-OBS is
considered a superior solution not only due to the
partial burst collision probability but also due to the
reduced switching node control and scheduling
complexity, when variable slot allocations need to
be scheduled in real-time. Therefore in this work,
the S-OBS is considered.
To limit the burst loss in large optical core
networks where dynamic resource allocation is
employed, CANON implements a hybrid reservation
mode. Electronic buffering is still employed at
network edge nodes, which are called Regular
Nodes (RNs) but optical frame generation is not
performed based on local RN queue status
information as is done in OBS nodes but based on a
MAC protocol executed between a Master Node
(MN) and all RNs of a sub-net called “cluster”. We
will briefly describe this principle of operation in the
remainder of this section and in the next section we
will demonstrate how this distributed switching
architecture leads to nearly optimal performance
results.
2.1 CANON Architecture
The CANON solution has previously described by
A. Stavdas et al. (2008) and by J. D. Angelopoulos
et al. (2007) and the principle of operation is now
summarized. CANON is organising the nodes of a
core network in clusters, mainly based on vicinity,
traffic, legacy infrastructure and administrative
criteria. This process creates a new hierarchy since
the core nodes are classified as Master Nodes (MN)
and Regular Nodes (RN) based on functionality
considerations and a differentiated traffic handling
policy (i.e. traffic destined to nodes of the same
cluster versus transit traffic destined to the nodes of
a distant cluster). The role of the MN is to
coordinate both inter and intra-cluster operations. In
the latter process, the RNs contribute fixed size
contiguous optical slots, which are marshalled into
appropriately sized frames destined for other
clusters, under the guidance of a reservation-based
MAC protocol. In addition, under MN’s supervision,
RNs use the same frame and wavelength to transport
traffic to the same destination cluster, so each ring is
effectively operating as a “distributed switch”.
Details on traffic aggregation, flexible bandwidth
allocation, robustness and resiliency are discussed
by J. D. Angelopoulos et al. (2007). Regarding inter-
cluster operation, a pre-provisioned scenario has
been analysed by J. D. Angelopoulos et al. (2007)
whilst a fully dynamic case based on one-way
reservations over the pan-European network has
been benchmarked by A. Stavdas, A.
Orphanoudakis, C. (T) Politi, A. Drakos and A. Lord
(2009).
Regarding CANON node architectures, the
proposed MN has been presented by A. Stavdas, C.
(T) Politi, T. Orphanoudakis and A. Drakos (2008).
It is a wavelength and a link modular architecture
allowing the node to gracefully scale to hundreds of
Tb/s. On the other hand, the RNs are optical
add/drop mulitplexers (OADMs). This solution
greatly supports the smooth migration from existing
rings to the CANON solution. Conclusively, the
combined operation of network and switch
architecture allows to efficiently groom slots into
frames in a collision free-way by means of a MAC.
3 BENCHMARKING CANON
3.1 Benchmarking Parameters
Since the performance of OBS is directly
proportional to the available number of wavelengths
STATISTICAL TRAFFIC MULTIPLEXING WITH SERVICE GUARANTEES OVER OPTICAL CORE NETWORKS
115