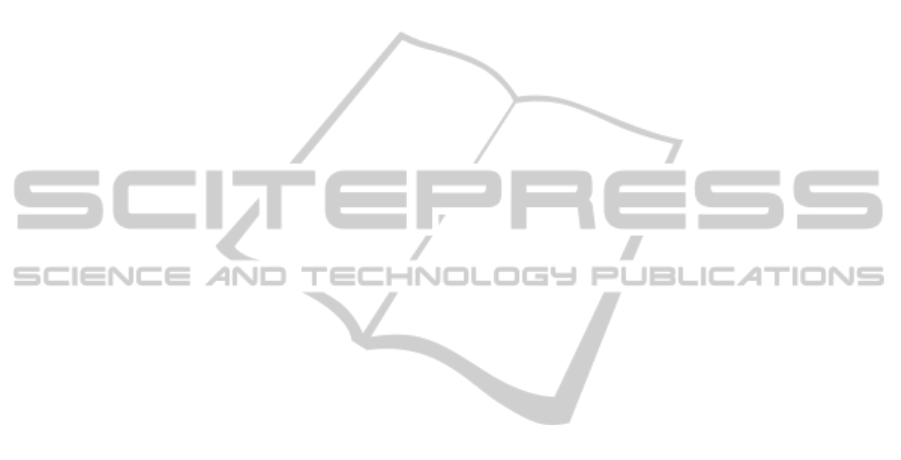
infection. The penetration of the photosensitizer
solution into the bacterial biofilm is decreased in
comparison to the suspensions of bacteria used in in
vitro studies. Therefore, to enhance the effectiveness
of PACT, the development of novel delivery and
targeting approaches may be required. One strategy
to improve the targeting was proposed by Bhatti et
al. (2000). The authors used a conjugate of TBO and
murine monoclonal antibody (Ab-TBO) to
specifically target P. gingivalis in the presence of S.
sanguis or human gingival fibroblasts (HGFs) in
vitro. It was demonstrated that with the use of Ab-
TBO conjugate a high selectivity and efficiency in
the killing of P. gingivalis can be achieved. Such an
approach could enable the killing of important
periopathogens without collateral damage either to
host tissues or to the normal oral microflora.
3 CONCLUSIONS
PDT and PACT are non-invasive, relatively
inexpensive, painless to the patient with little or no
side-effects. The outcomes of presented in vitro and
in vivo studies are very promising. However, more
research is still needed in this field for optimizing
the protocol of clinical application, improving
specific targeting of tumor cells and bacteria and
introducing new groups of photosensitizers.
REFERENCES
Albandar, J. M., (2005). Epidemiology and risk factors of
periodontal disease. Dental Clinics of North America,
49, 517-532.
Bahrami, G., Vaeth, M., Kirkevang, L. L., Wenzel, A.,
Isidor, F., (2008). Risk factor for tooth loss in an adult
population: radiographic study. Journal of Clinical
Periodontology, 35, 1059-1065.
Bhatti, M., MacRobert, A., Henderson, B., Shepherd, P.,
Cridland, J., Wilson, M., (2000). Antibody-targeted
lethal photosensitization of Porphyromonas gingivalis.
Antimicrobial Agents and Chemotherapy, 44, 2615-
2618.
Bhatti, M., MacRobert, A., Henderson, B., Wilson, M.,
(2002). Exposure of Porphyromonas gingivalis to red
light in the presence of the light-activated
antimicrobial agent toluidine blue decreases
membrane fluidity. Current Microbiology, 45: 118-
122.
Bonsor, S. J., Nichol, R., Reid, T. M. S., Pearson, G. J.,
(2005). An alternative regimen for root canal
disinfection. British Dental Journal, 201, 101-105
Bonsor, S. J., Nichol, R., Reid, T. M. S., Pearson, G. J.,
(2006). Microbiological evaluation of photo-activated
disinfection in endodontics (An in vivo study). British
Dental Journal, 200: 337-341.
Braun, A., Dehn, C., Krause, F., Jepsen, S., (2008). Short-
term clinical effects of adjunctive antimicrobial
photodynamic therapy in periodontal treatment: a
randomized clinical trial. Journal of Clinical
Periodontology, 35: 877-884.
Burns, T., Wilson, M., Pearson, G. J., (1995). Effect of
dentine and collagen on the lethal photosensitization
of Streptococcus mutans. Caries Research, 29(3): 192-
197
Chan, Y., Lai, C. H., (2003). Bactericidal effects of
different laser wavelength on periodontopathic germs
in photodynamic therapy. Lasers in Medical Science,
18(1), 51-55.
Chondros, P., Nikolidakis, D., Christodoulides, N.,
Roessler, R., Gutknecht, N., Sculean, A., (2009).
Photodynamic therapy as adjunct to non-surgical
treatment in patients on periodontal maintenance: a
randomized controlled clinical trial. Lasers in Medical
Science, 24: 681-688.
Detert, J., Pischon, N., Burmester, G. R., Buttgereit, F.,
(2010). The association between rheumatoid arthritis
and periodontal disease. Arthritis Research &
Therapy, 12: 218-224.
Fernandes, L. A., de Almeida, J. M., Theodoro, L. H.,
Bosco, A. F., Nagata, M. J. H., Martins, T. M.,
Okamoto, T., Garcia, V. G., (2009). Treatment of
experimental periodontal disease by photodynamic
therapy in immunosprressed rats. Journal of Clinical
Periodontology, 36: 219-228.
Foschi, F., Fontana, C. R., Ruggiero, K., Riani, R., Vera,
A., Doukas, A. G., Pagonis, T. C., Kent, R.,
Stashenko, P. P., Soukos, N. S., (2007). Photodynamic
inactivation of Enterococcus faecalis in dental root
canals in vitro. Lasers in Surgery and Medicine, 39:
782-787.
Haas, R., Baron, M., Doertbudak, O., Watzek, G., (2000).
Lethal photosensitization, autogenous bone and e-
PTFE membrane for the treatment of peri-implantitis:
preliminary results. The International Journal of Oral
& Maxillofacial Implants, 15: 374-382.
Koemerik, N., Nakanishi, H., MacRobert, A. J.,
Henderson, B., Speight, P., Wilson, M., (2003). In
vivo killing of Porphyromonas gingivalis by toluidine
blue-mediated photosensitization in an animal model.
Antimicrobial Agents & Chemotherapy, 47: 932-940.
Lagervall, M., Jansson, L., Bergstroem, J., (2003).
Systemic disorders in patients with periodontal
disease. Journal of Clinical Periodontology, 30: 293-
299.
Lin, H. P., Chen, H. M., Yu, C. H., Yang, H., Wang, Y. P.,
Chiang, C. P., (2010). Topical photodynamic therapy
is very effective for oral verrucous hyperplasia and
oral erythroleukoplakia. Journal of Oral Pathology &
Medicine, 39: 624-630.
Matevski, D., Weersink, R., Tenenbaum, H. C., Wilson,
B., Ellen, R. P., Lepine, G., (2003). Lethal
photosensitization of periodontal pathogens be a red-
filtered Xenon lamp in vitro. Journal of Periodontal
Research, 38: 428-435.
BIODEVICES 2012 - International Conference on Biomedical Electronics and Devices
194