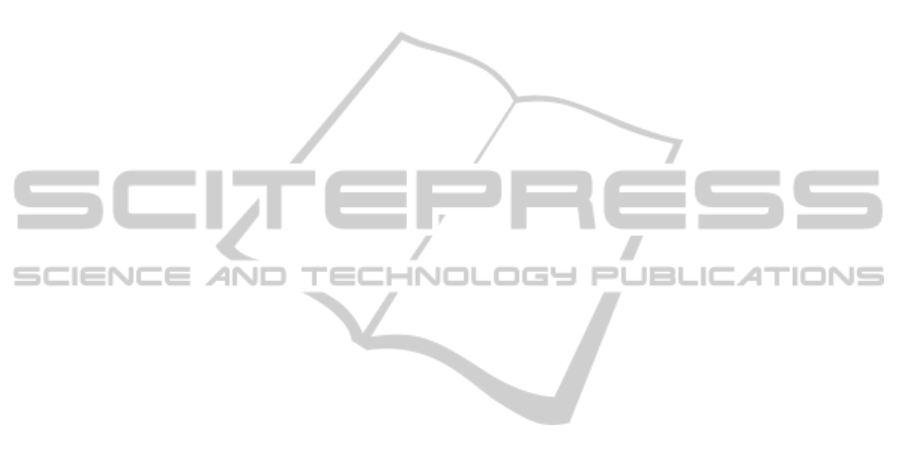
ACKNOWLEDGEMENTS
This work is a part of the project „System for Non-
invasive Detection of Glucose “supported by the
Foundation Baden-Württemberg Stiftung by
Research Program: Microsystem technology for the
life sciences.
REFERENCES
Abdallah O., Qananwah Q., Bolz, A. A., Hansmann J.,
Hinderer S., and Mertsching H., 2011. Fluorescence
Spectroscopy by Detection of Glucose Concentrations
in DMEM-Solutions and its Perspectives for Non-
invasive Measurement BIODEVICES 2011 part of
BIOSTEC, The International Joint Conference on
Biomedical Engineering Systems and Technologies,
Rome, Italy
Abdallah O., Hansmann J., Bolz A. and Mertsching H.,
2010. "IR spectroscopy vs. Raman scattering by
measurement of glucose concentration", Proc. SPIE
7376, 73760B; doi:10.1117/12.871469
Barman Ishan, Chae-Ryon Kong, Gajendra P. Singh,
Ramachandra R. Dasari, and Michael S. Feld, 2010:
Accurate Spectroscopic Calibration for Noninvasive
Glucose Monitoring by Modeling the Physiological
Glucose Dynamics. Analytical Chemistry 2010, Vol.
82, No. 14, pp. 6104–6114
Caduff, A., Talary, M., Mueller, M., Dewarrat, F., Klisic,
J., Donath, M., Heinemann, L., Stahel, W., 2009. Non-
invasive glucose monitoring in patients with Type 1
diabetes: A Multisensor system combining sensors for
dielectric and optical characterisation of skin. Bio-
sensors and Bioelectronics Vol. 24, pp. 2778– 2784.
D’Auria, S., Herman, P., Rossi, M., Lakowicz, J., 1999.
The Fluorescence Emission of the Apo-glucose
Oxidase from Aspergillusniger as Probe to Estimate
Glucose Concentrations. Biochemical and Biophysical
Research Communications, Vol. 263, pp. 550-553.
Evans, N., Gundi, L., Rolinski, O., Birch, D., Pickup, J.,
2003. Non-invasive glucose monitoring by NAD(P)H
autofluorescence spectroscopy in Fibroblasts and
adipocytes: A model for skin glucose sensing.
Diabetes Technology & Therapeutics, Vol. 5, No. 5.
Evans, N.D., Gnudi, L., Rolinski, O.J., Birch, D.J., Pickup,
J.C., 2005. Glucose-dependent changes in NAD(P)H-
related fluorescence lifetime of adipocytes and
fibroblasts in vitro: Potential for non-invasive glucose
sensing in diabetes mellitus. J. of Photochemistry and
Photobiology B: Biology, Vol. 80, pp. 122-129.
Gaster, Barak, Hirsch, MD; Irl B., MD, 1998. The Effects
of Improved Glycemic Controlon Complications in
Type 2 Diabetes, Arch Intern Med/VOL 158
Harman-Boehm Ilana, Avner Gal, Alexander M.
Raykhman, Jeffrey D. Zahn, Eugene Naidis, and
Yulia Mayzel, 2009. Noninvasive Glucose
Monitoring: A Novel Approach, Journal of Diabetes
Science and Technology Volume 3, Issue 2
Khalil, O.S., 2004. Non-invasive glucose measurement
technologies: An update from 1999 to the dawn of the
new millennium. Diabetes Technol. Ther., 6, 660-697
Lakovics, J., 2006. Principles of Fluorescence. Springer.
Maruo K., M. Tsurugi, J.Chin, T. Ota, H. Arimoto, Y.
Yamada, M. Tamura, M. Ishii, and Y. Ozaki, 2003.
"Noninvasive Blood Glucose Assay Using a Newly
Developed Near-Infrared System," IEEE Journal Of
Selected Topics In Quantum Electronics, 9 (2)
Mezzetti Andrea, Cipollone Francesco, Cuccurullo
Franco, 2000. Oxidative stress and cardiovascular
complications in diabetes: Isoprostanesas new markers
on an old paradigm, Cardiovasc. Research 47, 475–
488
Moschou, E., Sharma, P., Deo, S., Daunert, S., 2004.
Fluorescence Glucose Detection: Advances Toward
the Ideal In Vivo Biosensor. Journal of Fluorescence,
Vol. 14, No. 5.
Pambianco Georgia, Tina Costacou, Demetrius Ellis,
Dorothy J. Becker, Ronald Klein, andTrevor J.
Orchard, 2006. The 30-Year Natural History of Type 1
Diabetes Complications: The Pittsburgh Epidemiology
of Diabetes ComplicationsStudy Experience,
DIABETES, VOL. 55
Pickup, C. John, Faeiza Hussain, Nicholas D. Evans, Olaf
J. Rolinski, David J.S. Birch: Review: Fluorescence-
based glucose sensors, Biosensors and Bioelectronics
20, 2555–2565 (2005)
Smith John L., 2011, The Pursuit of Noninvasive Glucose:
“Hunting the Deceitful Turkey” Second Edition
Tura Andrea, Stefano Sbrignadello, Domenico
Cianciavicchia, Giovanni Pacini and Paolo Ravazzani,
2010. A Low Frequency Electromagnetic Sensor for
Indirect Measurement of Glucose Concentration: In
Vitro Experiments in Different Conductive Solutions
Sensors 10, pp. 5346-5358;
Wang Chuji and Sahay Peeyush, 2009, Breath Analysis
Using Laser Spectroscopic Techniques: Breath
Biomarkers, Spectral Fingerprints, and Detection
Limits, Sensors 2009, 9, PP. 8230-8262
Yamakoshi, K. Y. Yamakoshi: Pulse Glucometry, 2006. A
New Approach for Non-invasive Blood Glucose
Measurement Using Instantaneous Differential Near
Infrared Spectrophotometry, Journal of Biomedical
Optics 11(5), 1-11
Zierler, K., 1999. Whole body glucose metabolism. Am J
Physiol, 276(3 Pt 1):E409-E426
BIOSIGNALS 2012 - International Conference on Bio-inspired Systems and Signal Processing
296