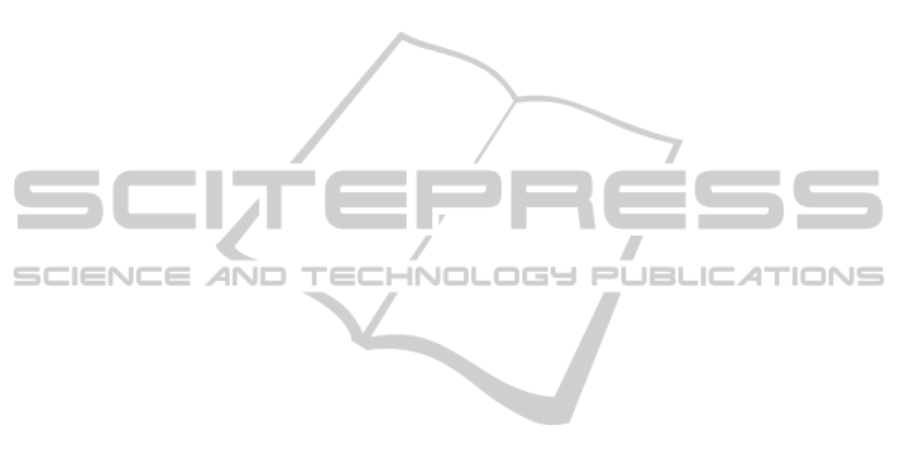
cultivated and uncultivated bare surfaces. Remote
Sensing of Environment, 90, 505–523.
Cierniewski, J. and Gdala T. (2010). Calculating the
optimal time when albedo approximates its daily
average: an example using soil surfaces with various
roughnesses at different latitudes. International Jour-
nal of Remote Sensing, 31, 2697-2708.
Cierniewski, J., Karnieli, A., Kuśnierek, K. and Herrmann,
I. (2012) Approximation the average daily surface
albedo with respect to soil roughness and latitude.
International Journal of Remote Sensing (accepted for
publication).
Coulson, K. L. (1966). Effect of reflection properties of
natural surfaces in aerial reconnaissance. Applied
Optics, 5, 905-917.
Eckardt, M. (1991). Albedo changes and satellite
observations of the Earth. In Climate and Global
change – Proceedings of the European School of
Climatology and Natural Hazards Course, held in
Arles/Rhone, France, from 4 to 12 April 1990.
Brussels, Lxemburg: ECSC-EEC-EAEC, 123-137.
Kondratyev, K. Y. (1969). Radiacjonnyje Charakteristiki
Atmosfery i Zemnoy Poverkhnosti. Leningrad: Gidro-
meteorologicheskoye Izdatelstvo.
Lewis, P. and Barnsley, M. J. (1994). Influence of the sky
radiance distribution on various formulations of the
Earth surface albedo. In Proceedings Mesures
Physiques et Signatures en Teledetection, Val d’Isere,
France, 17–21 January, 1994, 707–716.
Liang G, S., Strahler, A. H. and Walthall, C. (1999).
Retrieval of land surface albedo from satellite
observations: a simulation study. Journal of Applied
Meteorology, 38, 712–725.
Martonchik, J. V., Brugge, C. J. and Strahler, A. (2000). A
review of reflectance nomenclature used in remote
sensing. Remote Sensing of Environment, 19, 9–20.
Matthias, A. D., Fimbres, A., Sano, E. E., Post, D. F.,
Aciolly, L., Batchily, A, K., and Ferreira, L. G.
(2000). Surface roughness effects on soil albedo, Soil
Science Society of America Journal, 64: 1035-1041.
Maurer J. (2002). Retrieval of surface albedo from space.
Retrived from: http://www2.hawaii.edu/~jmaurer/.
Milton, E. J. and Webb, J. P. (1987). Ground radiometry
and airborne multispectral survey of bare soils.
International Journal of Remote Sensing, 18, 3-14.
Olsen K. W., Bonan, G. B., Schaaf, C., Gao, F., Jin, Y.
and Strahler, A. (2003). Assessment of global climate
model land surface albedo using MODIS data.
Geophysical Research Letters, 30, 1443, doi: 10.1029/
2002GL016749.
Pinty, B., Verstratte, M. M. and Dickinson, R. E. (1989).
A physical model for predicting bidirectional
reflectance over bare soil. Remote Sensing of
Environment, 27, 273–288.
Schaepman-Strub, G., Schaepman, M. E., Painter, T. H.,
Dangel, S. and Martonchik, J. V. (2006). Reflectance
quantities in optical remote sensing – definitions and
case studies. Remote Sensing of Environment, 45, 15–
27.
Shoshany, M. (1993). Roughness-reflectance relationship
of bare desert terrain; An empirical study. Remote
Sensing of Environment, 103, 27–42.
Sellers, P. J., Meeson, B. W., Hall, F. G., Asrar, G.,
Murphy, R. E., Schiffer, R. A., Bretherton, F. P.,
Dickinson, R. E., Ellingson, R. G., Field, C. B.,
Hummerich, K. F., Justice, C. O., Melack, J. M.,
Roulet, N. T., Schimel, D. S. and Try, P. D. (1995).
Remote Sensing of the land-surface for studies of
global change: models-algorithms-experiments.
Remote Sensing of Environment, 51, 3-26.
Sparks, D. L., Page, A. L., Helmke, P. A., Loeppert, R. H.,
Soltanpour, P. N., Tabatabai, M. A., Johnston, C. T.
and Summer, M. E. (1996). Methods of Soil Analysis.
Part 3. Madison: Soil Science Society of America and
American Society of Agronomy.
Tsvetsinskaya, E. A., Schaaf, C.B., Gao, F., Strahler, A.
H. and Dickinson, R. E. (2006). Spatial and temporal
variability in moderate resolution imaging
spectroradiometer derived surface albedo over global
arid regions. Journal of Geophysical Research, 111,
D20106, doi:10.1029/2005JD006772.
Wang, Z., Barlage, M. and Zeng, X. (2005). The solar
zenith angle dependence of desert albedo. Geophysical
Research Letters, 32, doi: 10.1029/2004GL021835.
Zhou, L., Dickinson, R. E., Tian, Y., Zeng, X., Dai, Y.,
Yang, Z.-L., Schaaf, C. B., Gao, F., Jin, Y., Strahler,
A., Myeneni, R. B., Yin, H., Wu, W. and Shaikh, M.
(2003). Comparison of seasonal and spatial variations
of albedos from Moderate-Resolution Imaging
Spectroradiometer (MODIS) and Common Land
Model. Journal of Geophysical Research, 108, 15-1-
15-20.
SENSORNETS 2012 - International Conference on Sensor Networks
234