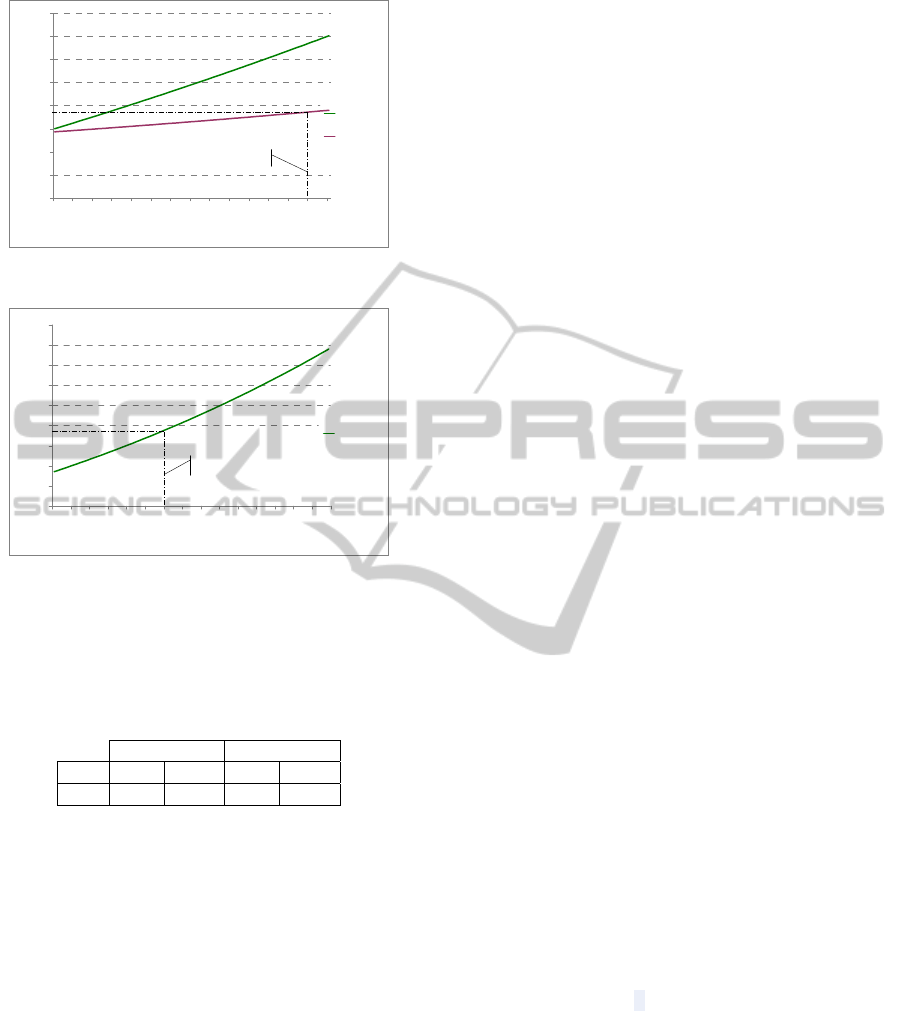
7,0
7,5
8,0
8,5
9,0
9,5
10,0
10,5
11,0
81
82
83
8
4
85
8
6
8
7
88
8
9
90
91
9
2
93
94
95
Flow (m3/h)
Eletric Power (kW)
n=2 e f=0
n=2 e f=1
CT case
Figure 5: CP – CT case.
3,0
3,5
4,0
4,5
5,0
5,5
6,0
6,5
7,0
7,5
5
0
5
2
5
4
56
58
6
0
6
2
6
4
6
6
68
7
0
72
7
4
7
6
7
8
Flow (m3/h)
Eletric Power (kW)
n=1 e f=0
RMP case
Figure 6: CP – RMP case.
For HVAC and PS the results for the power
reduction potentials are determined and presented in
Table 3.
Table 3: Potential power reduction (kW).
HVAC PS
CT 14.13 24.5% 29.41 42.9%
RMP 4.06 7.0% 14.3 20.9%
It is remarkable the enormous reduction of
power demand in the case of PS and the substantial
decrease in HVAC.
5 CONCLUSIONS
According to the present results the potential for
application of DR concept in this pool is important.
The BEMS designed by the author’s implements
some control strategies applied to HAVC system and
PS to reduce electricity demand during peak hours,
which represents a significant reduction in the power
demand of 7.0 % and 20.9% in the HVAC system
and the PS system, respectively. In a situation of
emergency to the grid, the maximum reduction in
power demand that can be obtained is 24.5 % and
42.9% in HVAC and PS, respectively.
It is expected a promising future for DR in these
kinds of buildings taking into account the large
number of such sport complexes in Portugal.
The authors believe that the present contribution
underlines the importance of sport complexes with
Indoor Swimming Pools for contract-based DR, of
using
adapted Building Energy Management System.
This work has been partially supported by FCT
under project grant PEst-C/EEI/UI0308/2011.
REFERENCES
Akayleh A., Mohammed A., Essam A., Salah A., 2009,
Water Pumping System with PLC and Frequency
Control, Journal of Mechanical and Industrial
Engineering, Pages 216 – 221,.
DHHS - Department of Health and Human Services, 2005,
Swimming pool and spa manual and operator’s
course, Nebraska Government.
EC - European Community, 2006, DIRECTIVE
2006/32/EC - Energy end-use efficiency and energy
services, Official Journal of the European Union.
EC - European Community, 2011, Smart Grids Task force
- Expert Group 3: Roles and Responsibilities of Actors
involved in the Smart Grids Deployment.
IPQ - Instituto Português da Qualidade, 2009, NP EN
15288-1:2009 - Piscinas Parte 1: Requisitos de
segurança para a concepção.
Jacopo, T., Mohamed, G., Matthew, L., 2009, Demand
response experience in Europe: Policies, programmes
and implementation, Energy doi:10.1016/j.energy.200
9.05.021.
Langelier, W., 1936, The analytical control of anti-
corrosion water treatment, Journal AWWA.
Pedrini, A., 2003, Integration of low energy strategies to
the early stages of design process of office buildings in
warm climate, Universidad de Queensland, Australia.
Perkins, P., 2000, Swimming Pools, Taylor & Francis e-
Library.
Ribeiro, E., Jorge, H., Quintela, D., 2010, A introdução de
VEV’s no sistema de bombagem de piscinas interiores,
em piscinas interiores, PCEEE - Portugal em
Conferência para uma Economia Energeticamente
Eficiente.
Ribeiro, E., Jorge, H., Quintela, D., 2011, HVAC system
energy optimization in indoor swimming pools, 3
rd
International Youth Conference on Energetic,
Rodrigues, P., 2007, Climatização de Recintos com
Piscinas Interiores – Eficiência Energética, Technical
Report.
Shah, M., 2003, Prediction of evaporation from occupied
indoor swimming pools, Energy and Buildings 35,
pages 707–713.
USDE – US Department of Energy, The Office of Energy
Efficiency and Renewable Energy, 2009, Swimming
Pool Cover.
SMARTGREENS2012-1stInternationalConferenceonSmartGridsandGreenITSystems
148