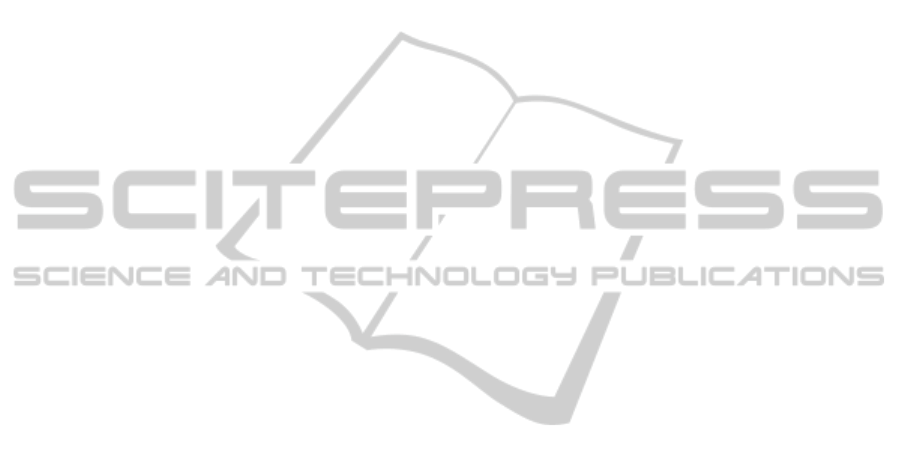
accident instantaneously and in real time.
It is also worth specifying that the analyses did
not consider any actual data on the use of the I2V
technologies – since they are not available to date –
or any active intervention on the vehicle in case of
need.
The subject is in evolution and many questions
remain open. For example an investigation of
vehicle behaviour, when it is not isolated in
approaching the traffic signal, need more tests,
possibly also with a traffic micro-simulation tool. It
can be assumed that the actual potential of the
systems which have been taken into consideration
could be assessed once said technologies are widely
spread on the market.
REFERENCES
Archer J., 2005. Indicators for traffic safety assessment
and prediction and their application in micro –
simulation modelling: A study of urban and suburban
intersections. Doctoral Thesis; Royal Institute of
Technology, Stockholm, Sweden.
Benz T., Gaitanidou E., Spyropoulou I., Yannis G., and
Tapani A.,2006. Modelling road traffic safety - The
In-Safety approach. In Proceedings of the 13th World
Congress and Exhibition on Intelligent Transport
Systems and Services, ERTICO, London.
COOPERS project CO-OPerativeSystEms for Intelligent
Road Safety - (accessed March 07, 2012)
http://www.coopers-ip.eu/index.php?id=project.
CoVeL - Cooperative Vehicle Localization for Efficient
Urban Mobility (accessed March 07, 2012)
http://www.covel-project.eu/.
CVIS Cooperative Vehicle-Infrastructure Systems
(accessed March 07, 2012)
http://www.cvisproject.org/.
Dalla Chiara B., Deflorio F. P., Diwan S., Assessing the
effects of inter-vehicle communication systems on
road safety, IET Intelligent Transport Systems, June
2009, IET Intell. Transp. Syst., 2009, Vol. 3, Iss. 2, pp.
225–235, doi: 10.1049/iet-its:20080059.
Day C. M., Premachandra H., Brennan T. M., Sturdevant
J. R., Bullock D. M., Operational Evaluation of
Wireless Magnetometer Vehicle Detectors at
Signalized Intersection, Transportation Research
Record 2192.
FHWA (2006). Traffic Detector Handbook: Third Edition
Chapter 4. http://www.fhwa.dot.gov (accessed March
07, 2012).
FOTsis - European Field Operational Test on Safe,
Intelligent and Sustainable Road Operation (accessed
March 07, 2012) http://www.fotsis.com/.
Gettman D. & Pu L., 2006. Theoretical validation of
Surrogate Safety Assessment Methodology for
roundabouts and cross intersections. In Proceedings of
the 13th World Congress and Exhibition on Intelligent
Transport Systems and Services, London.
Hurwitz, D. S., 2009. Application of Driver Behavior and
Comprehension to Dilemma Zone Definition and
Evaluation. Open Access Dissertations. Paper 112,
University of Massachusetts.
INTERSAFE-2 – Cooperative Intersection Safety
(accessed March 07, 2012) http://www.intersafe-
2.eu/public/
Johansson G., Rumar K., 1971. Driver's brake reaction
times. Human Factors, 13(1), pp. 23-27
Ki-Joon K., Jaehoon S., 2009. Development of
Intersection Traffic Accident Risk Assessment Model
- Application of Micro-simulation Model with SSAM
to Sungnam City. In IRTAD CONFERENCE, Seoul,
Korea.
Klunder G., Abdoelbasier A., Immers B., 2006.
Development of a micro-simulation model to predict
road traffic safety on intersections with surrogate
safety measures. In Proceedings of the 13th World
Congress and Exhibition on Intelligent Transport
Systems and Services, London.
Liu C., Herman R. and Gazis D.C., 1996. A review of the
yellow interval dilemma, Transportation Research Part
A: Policy and Practice, 1996, vol. 30, issue 5, pages
333-348.
MacNeill, P. Miller, R., 2003. A new technology for a
cruise control system A new technology for a cruise
control system. IEEE Vehicle Technology Conference
Maile M., Delgrossi L., 2009. Cooperative intersection
collision avoidance system for violations (CICAS-V)
for avoidance of violation-based intersection crashes.
Enhanced Safety of Vehicles.
Monteil J., Billot R., El Faouzi N. E., 2011. Towards
cooperative traffic management: methodological
issues and perspectives. In Australasian Transport
Research Forum 2011 Proceedings, 28 - 30 September
2011, Adelaide, Australia.
Morsink P. L. J., Wismans L. J. J., Dijkstra A., 2008.
Micro-simulation for road safety impact assessment of
advanced driver assistance systems. InEuropean ITS
Congress, Geneva.
Rausand M., Risk Assessment, 2011. Theory, Methods,
and Applications, Norwegian Univ. of Science and
Technology, ISBN: 978-0-470-63764-7, September
2011.
SAFESPOT Integrated Project (accessed March 07, 2012)
http://www.safespot-eu.org/.
Tapani A., 2009. Traffic simulation modelling of driver
assistance systems. In 16th World congress on ITS.
Torday A., Baumann D., Dumont A. G., 2003. Road
safety assessment using micro simulation based
indicators. Proc. of the ITS World Congress, Madrid.
U.S. Department of Transportation Federal Highway
Administration,2009.Surrogate Safety Assessment
Model (SSAM).
U.S. Department of Transportation, Cooperative
Intersection Collision Avoidance System Limited to
Stop Sign and Traffic Signal Violations (CICAS-V),
Task 8 Final Report, Prototype Build and Testing,
(Appendix F), June 24, 2008, August 29, 2008.
RoadSafetyatIntersectionsControlledbyTrafficLights-IVCandRiskIndexes
577