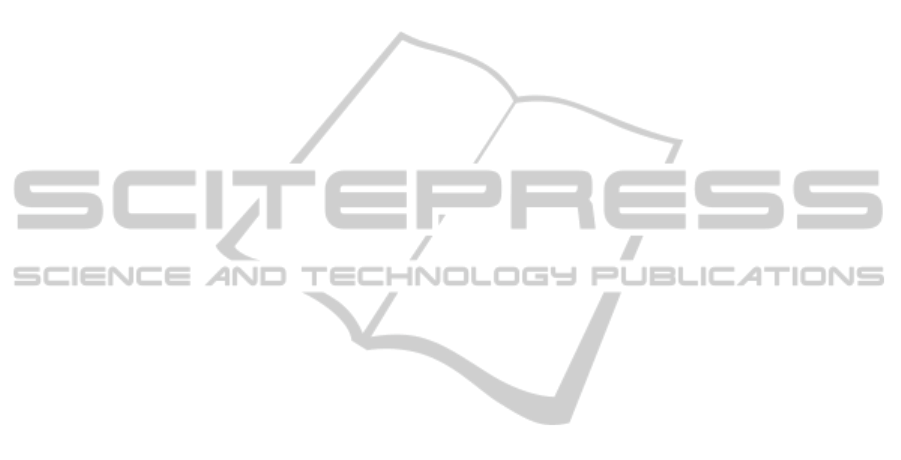
a clear initial reaction can be observed in the plot.
We speculate that this is more due to a transient
change in the breathing style than in the nasal
resistance. Following the short transition period, a
stable resistance curve follows which stays at the
same level as the baseline resistance.
4 CONCLUSIONS
A method to estimate continuous nasal airflow
resistance during a birch provocation test was
presented. The nasal resistance was estimated with a
new method that applies LMS filtering technique to
the nasal pressure signal and carefully calibrated
respiratory belt signals to update adaptively an
extended Broms model.
Quantitative results of resistance changes were
presented for two subject groups - birch pollen
allergic and non-allergic subjects - to demonstrate
their reactivity to the birch challenge. In the baseline
situation, the median resistance value was similar in
the groups. However, due to the birch challenge,
statistically significant changes in the individual
resistances were observed in allergic group, while no
statistically significant differences were observed in
the non-allergic group.
Continuous resistance curves were presented
from selected subjects to demonstrate the dynamic
changes in their nasal resistance during provocation
test. To our knowledge, this is the first time this kind
of dynamic resistance curves are presented for nasal
provocation tests.
Provocation tests like this one may cause
changes in the breathing style of subjects. This has
the undesired consequence of the fact that the
calibration model is not fully accurate all the time.
We are currently developing new adaptive
calibration methods to enhance the accuracy of flow
estimation for situations where the breathing style
changes.
Even at present, the method presented above
could improve the reliability and accuracy of
diagnostics and assessment of the effect of nasal
treatments.
ACKNOWLEDGEMENTS
We thank MD Aila Kristo for participating in the
collection of the data.
Finnish Cultural Foundation, North Ostrobothnia
Regional fund; Allergy Research Foundation; The
Research Foundation of the Pulmonary Diseases; and
The Finnish Research Foundation of Otology are
gratefully acknowledged for having provided
financial support for this work.
REFERENCES
Bousquet, J., Khaltaev, N., Cruz, A. A., Denburg, J.,
Fokkens, W. J., Togias A., et al., 2008. Allergic
Rhinitis and its Impact on Asthma (ARIA) 2008
Update (in collaboration with the World Healt
Organization, GA2LEN and AllerGen). Allergy,
63(Suppl 86), pp. 8-160.
Chaaban, M., and Corey, J. P., 2011. Assessing nasal air
flow: options and utility. Proceedings of the American
Thoracic Society, vol 8 March no. 1, pp. 70-78.
Hohlfeld, J.M., Holland-Letz, T., Larbig, M., Lavae-
Mokhtari, M., Wierenga, E., Kapsenberg, M., van Ree,
R., Krug, N., and Bufe, A., 2010. Diagnostic value of
outcome measures following allergen exposure in an
environmental challenge chamber compared with
natural conditions. Clinical & Experimental Allergy,
vol 40 issue 7, pp. 998-1006.
Kohler, M., Thurnheer, R., and Bloch, K. E., 2006. Side-
selective, unobtrusive monitoring of nasal airflow and
conductance. Journal of Applied Physiology, 101, pp.
1760-1765.
Naito, K., Iwata, S., Ohoka, E., Kondo, Y., and Taekuchi,
M., 1993. A comparison of current expressions of
nasal patency. European Archives of Oto-Rhino-
Laryngology, 250, pp. 249-252.
Pirilä, T., Talvisara, A., Alho, O-P, and Oja, H., 1997.
Physiological fluctuations in nasal resistance may
interfere with nasal monitoring in the nasal
provocation test. Acta Oto-laryngologica, 117, pp.
596-600.
Pirilä, T., and Nuutinen, J., 1998. Acoustic rhinometry,
rhinomanometry and the amount of nasal secretion in
the clinical monitoring of the nasal provocation test.
Clinical & Experimental Allergy, vol 28 issue 4, pp.
468-477.
Seppänen, T., Koskinen, M., Seppänen, T. M., and Alho,
O-P, 2009. Continuous assessment of nasal airflow
resistance by adaptive modeling. Physiological
Measurement, vol. 30, pp. 1197-1209.
Seppänen, T., Koskinen, M., Seppänen, T. M., and Alho,
O-P, 2010. Addendum to ´Continuous assessment of
nasal airflow resistance by adaptive modeling´ -
technical repeatability. Physiological Measurement,
vol. 31, pp. 1547-1551.
Seppänen, T. M., Alho, O-P, Koskinen, M., and Seppänen,
T., 2011. Improved calibration method of respiratory
belts by extension of multiple linear regression.
Proceedings of the 5
th
European Conference of the
International Federation for Medical and Biological
Engineering, 37:161-164.
Tobin, M., 1992. Breathing pattern analysis. Intensive
Care Medicine, 18, pp. 193-201.
BIOSIGNALS2013-InternationalConferenceonBio-inspiredSystemsandSignalProcessing
10