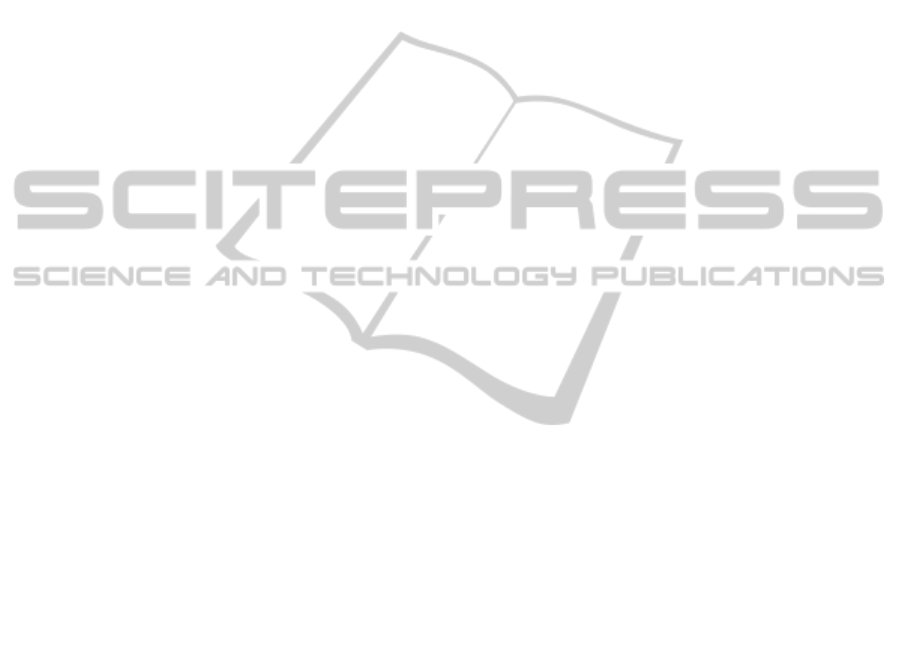
micromotor, the second one with a magnetic field
and the last one with an external tool. Our research
work consisted in evaluating the advantages and
drawbacks of each case. This led us to select the
micromotor approach as the most reliable one.
Different design solutions have been analysed
and discussed. We are currently developing an
original design of the actuated tibial implant, based
on the use of two rotary piezoelectric motors. This
model has been theoretically studied and simulations
on a detailed 3D CAD model proved its feasibility.
The 3D model optimisation stage will be followed
by the realization of a prototype, which will be
tested with a knee simulator. Results will be
presented in a future work.
REFERENCES
Almouahed, S., 2011. Etude, mise en oeuvre et évaluation
de prothèse autonome et prédictive du genou. Ph.D.
Télécom Bretagne - LaTIM INSERM U1101, Brest.
Almouahed, S., Gouriou, M., Hamitouche, C., Stindel, E.,
Roux, C., 2010. Design and evaluation of
Instrumented Smart Knee Implant. In IEEE
Transactions on Biomedical Engineering, vol. 58, n°
4, pp. 971–982.
Almouahed, S., Gouriou, M., Hamitouche, C., Stindel, E.,
Roux, C., 2011. The use of piezoceramics as electrical
energy harvesters within instrumented knee implant
during walking. In IEEE/ASME Transactions on
Mechatronics, Focused Section on Sensing
Technologies for Biomechatronics, vol. 16, pp. 799-
807.
Almouahed, S., Hamitouche, C., Stindel, E., Roux, C.,
2012. Finite Element Lifetime Prediction of a
Miniature Adjustable Orthopedic Device. In 34th
Annual International Conference of the IEEE
Engineering in Medicine and Biology Society
"Engineering Innovation in Global Health" -
EMBC'12.
Crottet, D., Maeder, T., Fritschy, D., Bleuler, H., Nolte, L.
P., Pappas, I. P., 2005. Development of a force
amplitude- and location-sensing device designed to
improve the ligament balancing procedure in TKA. In
IEEE Transactions on Biomedical Engineering, vol.
52, pp. 1609-1611.
International Organisation for Standardization, 2004. ISO
14243-3 Implants for surgery – Wear of total knee-
joint prostheses – Part 3: Loading and displacement
parameters for wear-testing machines with load
control and corresponding environmental conditions
for test.
Lahuec, C., Almouahed, S., Arzel, M., Hamitouche, C.,
Jézéquel, M., Stindel, E., Roux, C., 2010. A self-
powered telemetry system to estimate the
postoperative instability of a knee implant. In IEEE
Transactions on Biomedical Engineering, vol. 58, n°3,
pp. 822–825.
Marmignon, C., 2004. Modèle et instruments robotisés
pour l'étude de la biomécanique per-opératoire de
l'équilibre ligamentaire du genou. Ph.D. Université
Joseph Fourier, Grenoble.
Marmignon, C., Leimnei, A., Cinquin, P., 2004. Robotized
distraction device for knee replacement surgery. In
Proc. CARS, pp. 638-643.
Marmignon, C., Leimnei, A., Lavallée, S., Cinquin, P.,
2005. Automated hydraulic tensor for Total Knee
Arthroplasty. In Int J Med Robot, vol. 1, n
o
4, pp. 51-
57.
Scuderi, G. R. and Tria, A. J., 2006. Knee Arthroplasty
Handbook: Techniques in Total Knee and Revision
Arthroplasty, Springer. New York.
Vail, T. P., Lang, E., 2006. Surgical techniques and
instrumentation in Total Knee Arthroplasty. In: W. N.
Scott, Surgery of the Knee, Churchill Livingstone, pp.
1493-1498. New York.
Winemaker, M. J., 2002. Perfect balance in total knee
arthroplasty: the elusive compromise. In Journal of
Arthroplasty, vol. 17, n
o
1, pp. 2-10.
BIODEVICES2013-InternationalConferenceonBiomedicalElectronicsandDevices
102