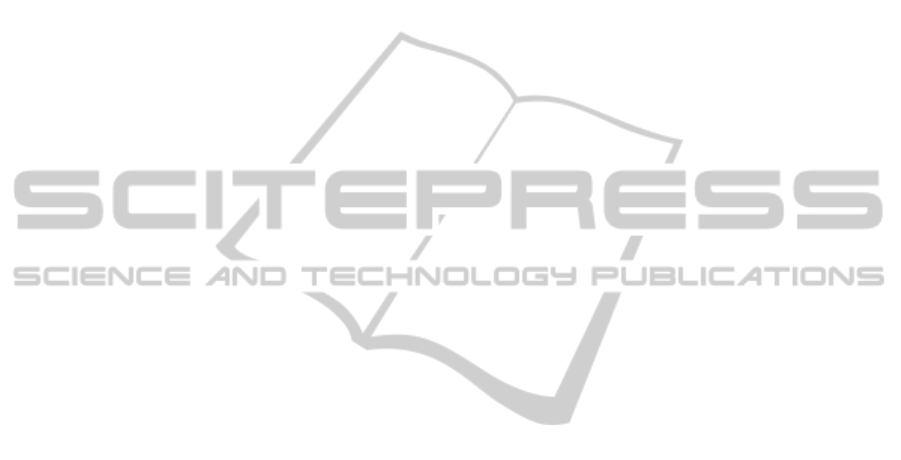
caused a decrease in perfusion, as was demonstrated
by a color test. Sersa et al., (2008) found that 3
minutes following tumor electric pulsing, blood flow
decreased by about 80 percent, and histological
evaluation of the endothelial cells showed that they
were rounded and swollen causing narrowing of the
blood vessels lumen.
Our study has several limitations to be
considered. First, blood pressure and pulse were not
measured or controlled during the experiments. This
could theoretically increase variance in the amount
of bleeding. Second, in this preliminary study we did
not address the effect of treatment in the case of
traumatic coagulopathy, which is expected in cases
of severe liver trauma. This issue calls for future
research. Other issues to be studied in larger animals
are the design of the electrodes in order to optimize
electric field geometry, optimize pulse parameters to
achieve finer results, better control of tissue
temperature, and the possible use of changes in the
electrical properties of the tissue for measuring
treatment effect.
In conclusion, in this preliminary research we
demonstrate that short electric pulses can
significantly reduce the amount of bleeding from
injured liver in a rat model. The effect is probably
non thermal and possibly related to the effect on
blood vessels’ endothelial layer. Further research is
needed in order to fully expose the potential if this
treatment modality for hemorrhage control in
civilian and military settings.
REFERENCES
Burgess, S., Zderic, V., & Vaezy, S. (2007). Image-guided
acoustic hemostasis for hemorrhage in the posterior
liver. Ultrasound in medicine & biology, 33(1), 113-9.
Gehl, J., Skovsgaard, T., & Mir, L. M. (2002). Vascular
reactions to in vivo electroporation: characterization
and consequences for drug and gene delivery.
Biochimica et biophysica acta, 1569(1-3), 51-8.
Graham, J., Bronskill, & Henkelman, (1998). Time and
Temperature Dependence of MR Parameters.pdf. MR
Parameters and Thermal Coagulation, 39, 198-203.
Guarini, S. (1971). Model of Arterial Thrombosis in Rats,
8719(96).
Hildreth, J., Wisner, D. H., Matsuoka, T. (1996).
Uncontrolled Hemorrhage from Parenchymal Injury :
Is Resuscitation Helpful ? The Journal of Trauma:
Injury, Infection, and Critical Care, 40(6), 915-922.
Hladovec J. (1975). A quantitative model of venous stasis
thrombosis in rats. Physiol Bohemoslov., 24(6), 551-4.
Holcomb, J. B., Pusateri, a E., Harris, R. a, Charles, N. C.,
Gomez, R. R., Cole, J. P., Beall, L. D., et al., (1999).
Effect of dry fibrin sealant dressings versus gauze
packing on blood loss in grade V liver injuries in
resuscitated swine. The Journal of trauma, 46(1), 49-
Kragh, John F., Jr, MC, U. C. M., Dubick, Michael A. ,
PhD David G. Baer, PhD James Johnson, P., &
Blackbourne, Lorne H. , MC, U., (2011). New
Tourniquet Device Concepts for Battlefield
Hemorrhage Control. THE ARMY MEDICAL
DEPARTMENT JOURNAL, 38-48.
Matsuoka, T., & Wisner, D. H., (1996). Resuscitation of
uncontrolled liver hemorrhage: effects on bleeding,
oxygen delivery, and oxygen consumption. The
Journal of Trauma: Injury, Infection, and Critical
Care, 41(3), 439-45.
Matsuoka, Ishida, M. and Konishi, (2004). Numerical
study of temperature distribution in tissue for
thermalcoagulation therapy..pdf. Journal of
Magnetism and Magnetic Materials, 272-276, 2426-
2427.
Matsushima Y, Takahashi E, Hagiwara K, Konaka C,
Miura H, Kato H, K. Y., (1994). Clinical and
experimental studies of anti-tumoral effects of
electrochemical therapy (ect) alone or in
combinationwith chemotherapy. Eur J Surgery,
574(59-67).
Palanker, D., Vankov, A., Freyvert, Y., & Huie, P.,
(2008). Pulsed electrical stimulation for control of
vasculature: temporary vasoconstriction and
permanent thrombosis. Bioelectromagnetics,
29(2),
100-7.
Precup, C. G., Gonganau-Nitu, D., Scurtu, R. R.,
Dindelegan, G., Biro, A., Soritau, O., Crişan, C., et al.,
(2010). Assessement by laser Doppler of the
peripheral tumour perfusion after radiofrequency
ablation for colorectal liver mestasis--experimental
study. Chirurgia (Bucharest, Romania : 1990), 105(1),
71-6.
Ramirez, Orlowski, Bindoula, Dzodic, Ardouin, Bognel,
Jr, B., et al. (1998). Electrochemotherapy on liver
tumours in rabbits. British Joumal of Cancer, 77(12),
2104-2111.
Sersa, G., Jarm, T., Kotnik, T., Coer, a, Podkrajsek, M.,
Sentjurc, M., Miklavcic, D., et al., (2008, January 29).
Vascular disrupting action of electroporation and
electrochemotherapy with bleomycin in murine
sarcoma. British journal of cancer.
Sersa, G. Parkins, C. . ., & Chaplin, D. J., (1999). Tumour
Blood Flow Changes Induced by Application of
Electric Pulses. European Journal of Cancer, 35(4),
672-677.
Vaezy, S., Martin, R., Schmiedl, U., Caps, M., Taylor, S.,
Beach, K., Carter, S., et al., (1997, January). Liver
hemostasis using high-intensity focused ultrasound.
Ultrasound in medicine & biology.
Yu-ling Xin, Fu-zhou Xue, Bing-sheng Ge, Feng-rui
Zhao, Bin Shi, and W. Z., (1997). Electrochemical
Treatment of Lung Cancer. Bioelectromagnetics, 18,
8-13.
HemorrhageControlbyShortElectricalPulses-InVivoExperiments
107