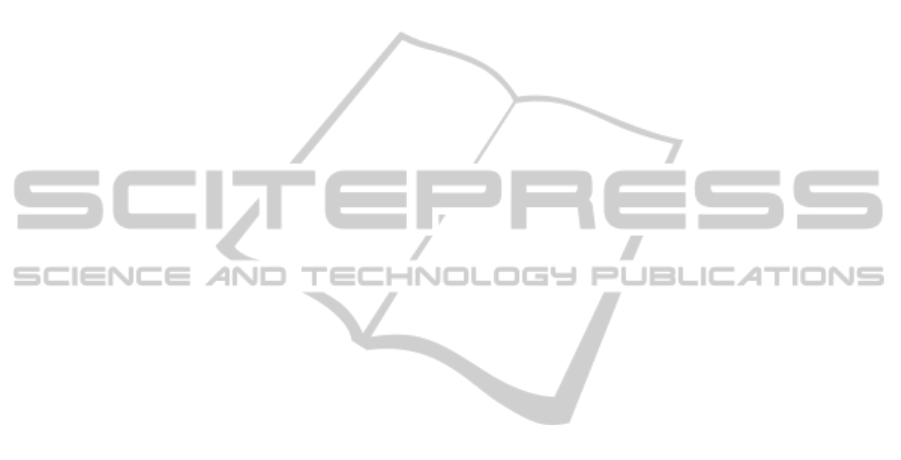
enhance the quality of non-invasive EEnG signals in
terms of electrocardiographic and respiratory
interferences, in comparison to bipolar recordings
with conventional disc electrodes. This is in
agreement with previous studies that used this kind
of electrodes implemented on rigid substrates (Prats-
Boluda, 2011). On one hand, the reduction of
respiratory interference permits to identify more
easily the activity of intestinal slow wave. On the
other hand, the reduction of ECG interference could
help the identification of spike bursts activity. This
could provide more robust systems to non-invasively
monitor intestinal myoelectrical activity which could
bring close the clinical application of this technique.
Nevertheless, this should be confirmed in future
studies.
Moreover, although it has not been tested in this
work, according to other authors (Besio and Chen,
2007); (Soundararajan and Besio, 2005) the
laplacian potential mapping can enhance spatial
sensibility for surface bioelectrical activity. This can
be of great importance for the studies of propagation
maps of cardiac, electroencephalographic or uterine
activity which can provide electrophysiological
information of clinical relevance. The developed
flexible array of active concentric ring electrodes
would be very suitable for these applications.
5 CONCLUSIONS
The flexible array of active concentric ring
electrodes developed in this paper joins the benefits
of laplacian techniques in terms of enhancing spatial
resolution, with the comfort and adaptation to body
surface curvature of conventional disposable
electrodes.
The non-invasive recordings of intestinal
myolectrical signals with this new kind of electrodes
provide enhanced bioelectric signals in terms of
robustness to physiological interferences such as
ECG and respiration, and permit to identify more
easily the intestinal slow wave activity.
ACKNOWLEDGEMENTS
Research supported in part by the Ministerio de
Ciencia y Tecnología de España (TEC 2010-16945)
and by Universitat Politènica de València (PAID
2009/10-2298).
REFERENCES
Besio, W., Aakula, R., Koka, K., and Dai, W., 2006.
Development of a tri-polar concentric ring electrode
for acquiring accurate Laplacian body surface
potentials. Ann.Biomed.Eng 34 426-435
Besio W., and Chen T., 2007. Tripolar Laplacian
electrocardiogram and moment of activation
isochronal mapping. Physiol, Meas. 28 515-529.
Bradshaw, L. A., Richards, W. O., and Wikswo, J. P., Jr.,
2001. Volume conductor effects on the spatial
resolution of magnetic fields and electric potentials
from gastrointestinal electrical activity. Med. Biol.
Eng. Comput. 39 35-43
Chang S. J., Cho E. T., Heo G. S., Park, C. G., Kim M.
W., Chang I. Y., Shin M. K., Cha, K. H., Yeum, C. H.,
Jun, J. Y., 2006. Fasting and postprandial small
intestinal slow waves non-invasively measured in
subjects with total gastrectomy. Gastroenterology
247-252.
Chen, J. D., Schirmer, B. D. and Mccallum, R. W., 1993.
Measurement of Electrical-Activity of the Human
Small-Intestine Using Surface Electrodes. IEEE Trans.
Biomed. Eng. 40 6 598-602.
Fleckenstein P. and Oigaard A., 1978. Electrical spike
activity in the human small intestine. A multiple
electrode study of fasting diurnal variations.
Am.J.Dig.Dis. 23: 776-780.
Garcia-Casado J., Martinez-de-Juan J. L., Ponce J. L.,
2006. Adaptive filtering of ECG interference on
surface EEnGs based on signal averaging. Physiol
Meas. 27: 509-527.
Hjorth, B., 1975. An on-line transformation of EEG scalp
potentials into orthogonal source derivations
Electroencephalogr. Clin. Neurophysiol. 39 526-530
Koka, K. and Besio, W. G., 2007. Improvement of spatial
selectivity and decrease of mutual information of tri-
polar concentric ring electrodes. J.Neurosci. Methods
165 216-222.
Li, G., Wang, Y., Jiang, W., Wang, L. L., Lu, C-Y S., and
Besio, W. G., 2005. Active Laplacian Electrode for the
Data-acquisition System of EHG. Journal of Physics:
Conference Series, 13, 330-335.
Lu, C. C. and Tarjan, P. P. 1999. An ultra-high common-
mode rejection ratio (CMRR) AC instrumentation
amplifier for laplacian electrocardiographic
measurement. Biomed. Instrum. Technol. 33 76-83.
Perrin, F., Pernier, J., Bertrand, O., Giard, M. H., and
Echallier, J. F. 1987. Mapping of scalp potentials by
surface spline interpolation. Electroencephalogr. Clin.
Neurophysiol. 66 75-81.
Prats-Boluda, G., Garcia-Casado, J., Martinez-de-Juan, J.
L., and Ponce J. L. 2007. Identification of the slow
wave component of the electroenterogram from
Laplacian abdominal surface recordings in humans.
Physol. Meas 28 1115-1133.
Prats-Boluda, G., Garcia-Casado, J., Martinez-de-Juan, J.
L., and Ye-Lin, Y. 2011. Active concentric ring
electrode for non-invasive detection of intestinal
myoelectric signals. Med.Eng Phys. 33 446-455.
BIODEVICES2013-InternationalConferenceonBiomedicalElectronicsandDevices
26