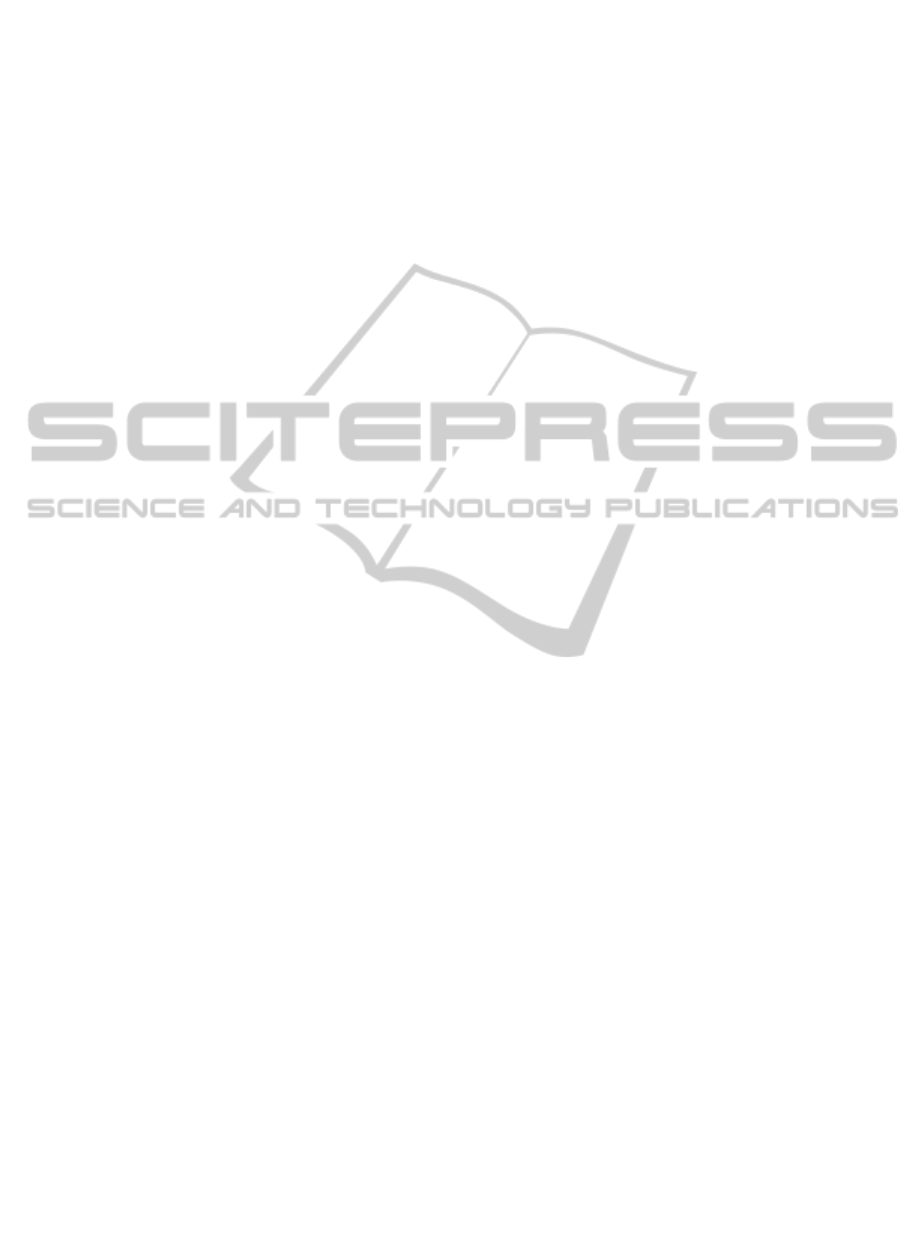
implemented in the project so far (KinectTM system
and VTE monitor) is relatively low cost and is under
continuous development by the manufacturers.
Furthermore, it offers flexibility in adjusting and
updating the interface to the changing needs of
patients (for example, partial recovery). Finally, the
CogWatch system is targeted at home-based
rehabilitation of the patients in a chronic phase of
the disease, when often they do not have further
access to rehabilitation from their medical providers.
The CogWatch system will deliver affordable and
easy access to therapeutic intervention at patients’
homes, which is the most comfortable and natural
setting, without a need for supervision.
An additional benefit for the patient is that the
Cogwatch system will be able to provide an online
and objective assessment of the patient’s progress
that can partially replace the clinic visits
(Giachristis, & Randall; submitted). In sum, the
CogWatch system will be driven by readily available
and cost efficient technology that can be customised
to patient requirements in order to heighten user-
friendliness.
ACKNOWLEDGEMENTS
This work was funded by the EU STREP Project
CogWatch (FP7-ICT- 288912).
REFERENCES
Amblard, B., Cremieux, J., Marchand, A. R., & Carblanc,
A. (1985). Lateral orientation and stabilization of
human stance: Static versus dynamic visual cues.
Experimental Brain Research, 61(1), 21-37.
Bickerton, W., Riddoch, J., Samson, D., Balani,A., Mistry,
B., & Humphreys, G., (2012). Systematic assessment
of apraxia and functional predictions from the
Birmingham Cognitive Screen, Journal of neurology,
neurosurgery & psychiatry with practical neurology,
83 (5), 513-52.
Christensen, A., Ilg, W., & Giese, M. A. (2011).
Spatiotemporal tuning of the facilitation of biological
motion perception by concurrent motor execution. The
Journal of Neuroscience, 31(9), 3493-3499.
Cooper, R. P.; Schwartz, M.; Yule, P. & Shallice, T.
(2005). The simulation of action disorganisation in
complex activities of daily living. Cognitive
Neuropsychology 22 (8) 959-1004.
De Renzi, E., Faglioni, P., & Sorgato, P. (1982) Modality
specific and supramodal mechanisms of apraxia. Brain,
105, 301–312.
Fadiga, L., Fogassi, L., Pavesi, G., & Rizzolatti G. (1995)
Motor facilitation during action observation: a
magnetic stimulation study, Journal of
Neurophysiology, 73, 2608–2611.
Gallese,V., & Goldman, A., (1998). Mirror neurons and
the simulation theory of mind-reading, Trends in
Cognitive Sciences, 2(12), 493-501.
Giachristis, C., & Randall, G. (submitted) CogWatch:
Cognitive Rehabilitation for Apraxia and Action
Disorganization Syndrome Patients. 6
th
International
Conference of Health Informatics.
Gibson, J. J. (1950). Perception of the visual world.
Boston: Houghton Mifflin.
Goldenberg, G., Hermsdörfer, J., Glindemann, R., Rorden,
C., & Karnath, H. O. (2007). Pantomime of tool use
depends on integrity of left inferior frontal cortex.
Cerebral Cortex, 17, 2769-2776.
Goldenberg, G., & Hagmann, S. (1998) Therapy of
activities of daily living in patients with apraxia.
Neuropsychological Rehabilitation, 8(2), 123–41.
Goldenberg, G., Hermsdörfer, J., & Spatt, J. (1996).
Ideomotor apraxia and cerebral dominance for motor
control. Cognitive Brain Research, 3, 95-100.
Goodale, M. A., & Keith Humphrey, G. (1998). The
objects of action and perception. Cognition, 67(1-2),
181-207.
Grassi, M. (2005). Do we hear size or sound? balls
dropped on plates. Attention, Perception, &
Psychophysics, 67(2), 274-284.
Gross, R., & Grossman, M. (2008). Update on apraxia.
Current neurology and neuroscience reports, 8 (6),
490-496.
Hazell, A. (2012) CogWatch – Cognitive Rehabilitation of
Apraxia and Action Disorganisation Syndrome:
Assessing the requirements of Healthcare
Professionals, Stroke Survivors and Carers. UK Stroke
Forum Conference 2012 Proceedings.
Hanna-Pladdy, B., Heilman, K.M., & Foundas, A.L.
(2003) Ecological implications of ideomotor apraxia:
evidence from physical activities of daily living.
Neurology, 60, 487–490.
Hermsdörfer, J., Hentze, S., & Goldenberg, G. (2006)
Spatial and kinematic features of apraxic movement
depend on the mode of execution. Neuropsychologia,
44, 1642–1652.
Hommel, B., Müsseler, J., Aschersleben, G., & Prinz, W.
(2001). The theory of event coding (TEC): A
framework for perception and action planning.
Behavioral and Brain Sciences, 24(05), 849.
Horstink, M., De Swart, B., Wolters, E., & Berger, H.
(1993). Paradoxical behavior in Parkinson's disease.
In: E. C Wolters, & P. Scheltens (Eds). Mental
dysfunction in Parkinson's disease; proceedings of the
European congress on mental dysfunction in
Parkinson's disease. Amsterdam: Vrije Universiteit,
1993. Proceedings of the European Congress on
Mental Dysfunction in Parkinson's Disease.
Houben, M., Kohlrausch, A., & Hermes, D. (2005). The
contribution of spectral and temporal cues to the
auditory perception of size and speed of rolling balls.
Acta Acustica United with Acustica, 91(6), 1007.
HEALTHINF2013-InternationalConferenceonHealthInformatics
226