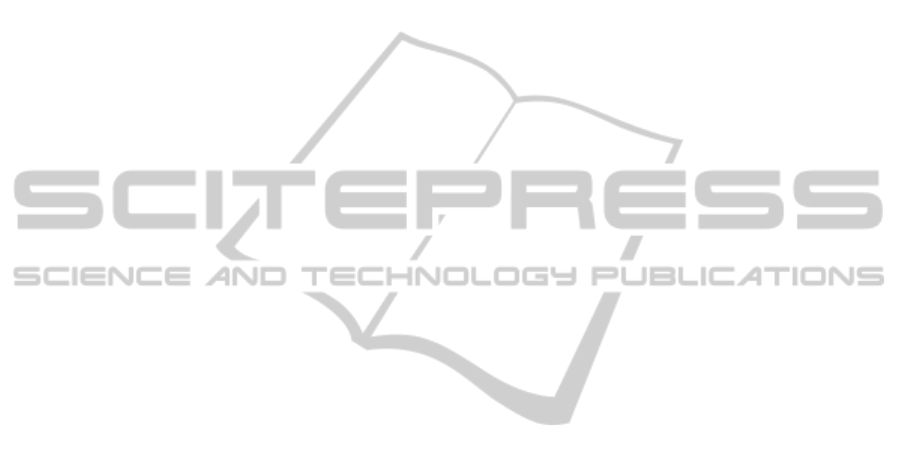
organization that defines, certifies and supports
enabling wireless technology for multimedia
applications, defined data rates up to 1 Gbit/s and
the creation of a global UWB radio standard with
guaranteed inter-operability as main targets for the
UWB radio specifications. Hence, UWB technology
presents the advantage of broadcasting higher data
rate between electronic devices than traditional
communications and it is indicated to be used by
high quality multimedia equipment as personal
digital video recorders (DVR), high definition
television (HDTV), laptops and cable/satellite set-
top boxes (ITU-R, 2004); (Wimedia, 2013).
The transmission of UWB radio signals along
optical fibre in short-range environments was
already investigated (Guo et al., 2007). The
application target of such investigation is to cover
buildings/offices with an integrated optical fibre
distribution/wireless broadcasting solution that
allows the end-users to benefit from the high
mobility and high bit-rate over short ranges
capabilities provided by the UWB-based wireless
networks. The transmission of these UWB radio
signals over fibre-to-the-home (FTTH)
infrastructures is a powerful solution to address the
distribution of UWB signals along longer distances.
Moreover, avoiding trans-modulation or frequency
conversion of the UWB radio signals at the end-
users' premises, the subscribers can benefit from low
cost transponders and a deep penetration can be
expected worldwide. The considered FTTH paths
correspond to standard lengths used in passive
optical networks (PONs) to connect distribution
hubs (DH) to UWB end users (up to 60 km).
Recently, the increase of the reach of the FTTH
paths has been a hot research topic supported by the
operators’ point of view (Davey et al., 2009). The
main target of this topic is to reach 100 km between
the central office and the users' premises, and it is
indicated for the metro and access networks
integration envisaged by long-reach (LR) PONs
(Davey et al., 2009). In LR-PONs, the optical line
termination (OLT) at the central office (CO) is
connected to an active remote node (RN) via a fibre
span denominated feeder or trunk line (Davey et al.,
2009). The target reach of this span is around 80 km
and optical amplification is performed at the RN in
order to compensate for the losses introduced along
the optical link. The different optical network units
(ONUs) are then connected to the RN via a
completely PON with reach around 20-30 km. From
the operators’ viewpoint, some of the benefits of the
LR-PONs are (Davey et al., 2009): i) decrease the
number of OLTs deployed and provide a full
integration between the metro and access networks
with the corresponding system cost savings, ii)
sharing the OLT and the feeder fibre by several
users in a sparse take-up geography and iii) decrease
the configuration and management issues of the
network.
External and direct modulation have been
recently proposed and demonstrated as effective
solutions to be employed in LR-PONs supporting
radio-over-fibre signals (Alves et al., 2013). External
modulated LR-PONs have shown better
performance, whereas directly modulated LR-PONs
are viewed as a cost-effective and alternative
solution. However, the maximum reach of directly
modulated LR-PONs is commonly assumed shorter
than when external modulation is employed due to
the combined effect of the chirp introduced by the
directly modulated laser (DML) and the fibre
dispersion.
The study of the directly modulated LR-PON
work proposed in (Alves et al., 2013) for the
provisioning of different wired and wireless OFDM-
based services to the end users showed that the
performance of the bundle of OFDM signals is
impaired mainly by the UWB signals. This is due to
the higher bandwidth of UWB signals and also due
to their higher central frequencies (Alves et al.,
2013).
In this work, we focus our attention only in the
transmission of the UWB signals along directly
modulated LR-PONs. Particularly, we propose and
demonstrate experimentally useful system design
guidelines enabling the distribution of UWB signals
along directly modulated LR-PONs with maximum
reach exceeding 100 km. This directly modulated
extended LR-PON is a powerful solution to
distribute the UWB signals to users’ premises
located at sparse geographical areas in a cost-
effective manner.
2 EXPERIMENTAL SETUP
Figure 1 depicts the diagram of the experimental
setup employed to assess the performance of the
OFDM-UWB signals distribution along the directly
modulated LR-PON. Figure 1 depicts a multi-
wavelength setup comprising three optical channels.
However, in this section, we focus the system
description only on the single-channel operation, i.
e., the switch S presented in Figure 1 is in the open
position. Further information about the multi-
wavelength setup is provided in section 3.2.
The OFDM-UWB bands #1, #2 and #3 are
generated and frequency multiplexed through digital
ExperimentalDistributionofOFDM-UWBRadioSignalsalongDirectlyModulatedLong-reachPonsIndicatedforSparse
GeographicalAreas
413