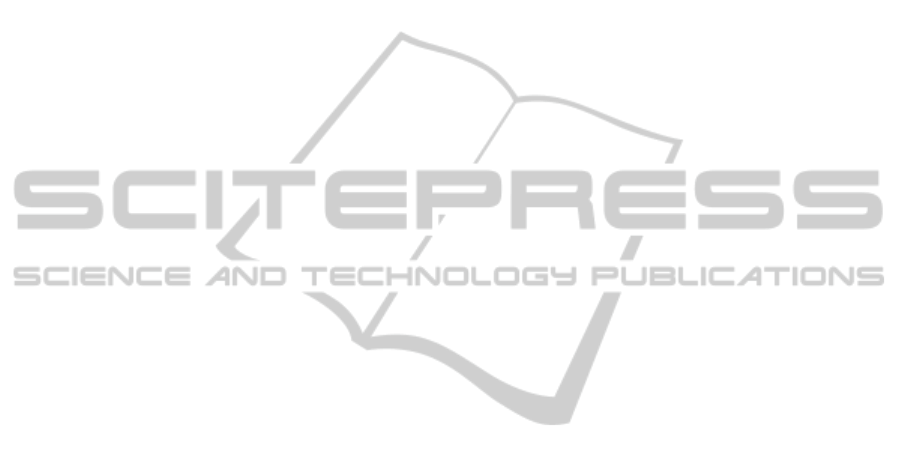
K. D., Moulton, M. J., Ratcliffe, M. B., Pasque, M. K.,
2001. Mechanism underlying mechanical dysfunction
in the border zone of left ventricular aneurysm: a finite
element model study. Ann Thorac Surg 71:654-662.
Hayashida, W., Kumada, T., Nohara, R., Tanio, H.,
Kambayashi, M., Ishikawa, N., Nakamura, Y.,
Himura, Y., Kawai, C., 1990. Left ventricular regional
wall stress in dilated cardiomyopathy. Circulation
82:2075-2083.
Jhun, C. S., Wenk, J. F., Zhang, Z., Wall, S. T., Sun, K.,
Sabbah, H. N., Ratcliffe, M. B., Guccione, J. M.,
2010. Effect of adjustable passive constraint on the
failing left ventricle: a finite-element model study.
Ann Thorac Surg 89:132-137.
McCulloch, A., Waldman, L., Rogers, J., Guccione, J.,
1992. Large-scale finite element analysis of the
beating heart. Crit Rev Biomed Eng 20:427-449.
Mirhosseini, S. M., Fakhri, M., Mozaffary, A., Lotfaliany,
M., Behzadnia, N., Ansari Aval, Z., Ghiasi, S. M.,
Boloursaz, M. R., Masjedi, M. R., 2013. Risk factors
affecting the survival rate in patients with
symptomatic pericardial effusion undergoing surgical
intervention. Interact Cardiovasc Thorac Surg 16:495-
500.
Nakayama, Y., Shimizu, G., Hirota, Y., Saito, T., Kino,
M., Kitaura, Y., Kawamura, K., 1987. Functional and
histopathologic correlation in patients with dilated
cardiomyopathy: an integrated evaluation by
multivariate analysis. J Am Coll Cardiol 10:186-192.
Omens, J. H., MacKenna, D. A., McCulloch, A. D., 1993.
Measurement of strain and analysis of stress in resting
rat left ventricular myocardium. J Biomech 26:665-
676.
Quarterman, R. L., Moonly, S., Wallace, A. W., Guccione,
J., Ratcliffe, M. B., 2002. A finite element model of
left ventricular cellular transplantation in dilated
cardiomyopathy. ASAIO J 48:508-513.
Ratcliffe, M. B., Hong, J., Salahieh, A., Ruch, S., Wallace,
A. W., 1998. The effect of ventricular volume
reduction surgery in the dilated, poorly contractile left
ventricle: a simple finite element analysis. J Thorac
Cardiovasc Surg 116:566-577.
Swynghedauw, B., 1999. Molecular mechanisms of
myocardial remodeling. Physiol Rev 79:215-262.
Vetter, F. J., McCulloch, A. D., 2000. Three-dimensional
stress and strain in passive rabbit left ventricle: A
model study. Ann Biomed Eng 28:781-792.
Walker, J. C., Ratcliffe, M. B., Zhang, P., Wallace, A. W.,
Fata, B., Hsu, E. W., Saloner, D., Guccione, J. M.,
2005. MRI-based finite-element analysis of left
ventricular aneurysm. Am J Physiol-Heart C
289:H692-H700.
Wall, S. T., Walker, J. C., Healy, K. E., Ratcliffe, M. B.,
Guccione, J. M., 2006. Theoretical impact of the
injection of material into the myocardium - A finite
element model simulation. Circulation 114:2627-2635.
Wenk, J., Jhun, C.-S., Sun, K., Ratcliffe, M., Guccione, J.,
2010. Surgical Left Ventricular Remodeling
Procedures, in: Guccione, J. M., Kassab, G. S.,
Ratcliffe, M. B. (Eds.), Computational Cardiovascular
Mechanics. Springer US, pp. 197-210.
Zhong, L., Su, Y., Yeo, S. Y., Tan, R. S., Ghista, D. N.,
Kassab, G., 2009. Left ventricular regional wall
curvedness and wall stress in patients with ischemic
dilated cardiomyopathy. Am J Physiol Heart Circ
Physiol 296:H573-584.
SIMULTECH2013-3rdInternationalConferenceonSimulationandModelingMethodologies,Technologiesand
Applications
644