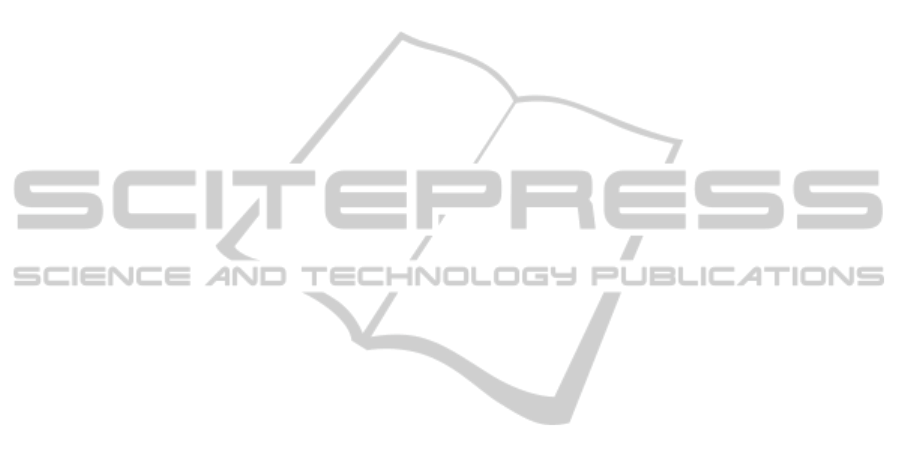
REFERENCES
Bergmann, T. O., M¨olle, M., Schmidt, M. A., Lindner, C.,
Marshall, L., Born, J., and Siebner, H. R. (2012).
EEG-Guided Transcranial Magnetic Stimulation Re-
veals Rapid Shifts in Motor Cortical Excitability dur-
ing the Human Sleep Slow Oscillation. Journal of
Neuroscience, 32(1):243–253.
Brugger, D., Butovas, S., Bogdan, M., and Schwarz,
C. (2011). Real-time adaptive microstimulation in-
creases reliability of electrically evoked cortical po-
tentials. IEEE transactions on bio-medical engineer-
ing, 58(5):1483–91.
Casarotto, S., Romero Lauro, L. J., Bellina, V., Casali,
A. G., Rosanova, M., Pigorini, A., Defendi, S., Mari-
otti, M., and Massimini, M. (2010). EEG responses
to TMS are sensitive to changes in the perturba-
tion parameters and repeatable over time. PloS one,
5(4):e10281.
Fadiga, L., Buccino, G., Craighero, L., Fogassi, L., Gallese,
V., and Pavesi, G. (1999). Corticospinal excitability is
specifically modulated by motor imagery: a magnetic
stimulation study. Neuropsychologia, 37(2):147–58.
Ferreri, F., Pasqualetti, P., M¨a¨att¨a, S., Ponzo, D., Fer-
rarelli, F., Tononi, G., Mervaala, E., Miniussi, C., and
Rossini, P. (2011). Human brain connectivity during
single and paired pulse transcranial magnetic stimula-
tion. NeuroImage, 54(1):90–102.
Hummel, F. and Cohen, L. (2006). Non-invasive brain stim-
ulation: a new strategy to improve neurorehabilitation
after stroke? The Lancet Neurology, 5(8):708–712.
Liepert, J., Bauder, H., Miltner, W., Taub, E., and Weiller,
C. (2000). Treatment-induced cortical reorganization
after stroke in humans. Stroke, 31(6):1210–1216.
Lioumis, P., Kici´c, D., Savolainen, P., M¨akel¨a, J. P.,
and K¨ahk¨onen, S. (2009). Reproducibility of TMS-
Evoked EEG responses. Human brain mapping,
30(4):1387–96.
Massimini, M., Ferrarelli, F., Huber, R., Esser, S. K.,
Singh, H., and Tononi, G. (2005). Breakdown of
cortical effective connectivity during sleep. Science,
309(5744):2228–32.
Matsumoto, R., Nair, D. R., LaPresto, E., Najm, I., Binga-
man, W., Shibasaki, H., and L¨uders, H. O. (2004).
Functional connectivity in the human language sys-
tem: a cortico-cortical evoked potential study. Brain,
127(Pt 10):2316–30.
Mitchell, K., Baker, M. R., and Baker, S. N. (2007). Muscle
responses to transcranial stimulation in man depend
on background oscillatory activity. Journal of Physi-
ology, 583(Pt 2):567–579.
Morishima, Y., Akaishi, R., Yamada, Y., Okuda, J., Toma,
K., and Sakai, K. (2009). Task-specific signal trans-
mission from prefrontal cortex in visual selective at-
tention. Nature Neuroscience, 12(1):85–91.
Nikulin, V. V., Kici´c, D., K¨ahk¨onen, S., and Ilmoniemi,
R. J. (2003). Modulation of electroencephalographic
responses to transcranial magnetic stimulation: evi-
dence for changes in cortical excitability related to
movement. The European Journal of Neuroscience,
18(5):1206–12.
Pfurtscheller, G. and Lopes da Silva, F. H. (1999). Event-
related EEG/MEG synchronization and desynchro-
nization: basic principles. Clinical Neurophysiology,
110(11):1842–57.
Plow, E. B., Carey, J. R., Nudo, R. J., and Pascual-Leone,
A. (2009). Invasive cortical stimulation to promote
recovery of function after stroke: a critical appraisal.
Stroke, 40(5):1926–31.
Ramos-Murguialday, A., Broetz, D., Rea, M., L¨aer, L., Yil-
maz, O., Brasil, F. L., Liberati, G., Curado, M. R.,
Garcia-Cossio, E., Vyziotis, A., Cho, W., Agostini,
M., Soares, E., Soekadar, S., Caria, A., Cohen, L. G.,
and Birbaumer, N. (2013). Brain-machine-interface in
chronic stroke rehabilitation: A controlled study. An-
nals of neurology, (Accepted).
Schulz, H., Ubelacker, T., Keil, J., M¨uller, N., and Weisz,
N. (2013). Now I am Ready–Now I am not: The In-
fluence of Pre-TMS Oscillations and Corticomuscu-
lar Coherence on Motor-Evoked Potentials. Cerebral
cortex.
Tsubokawa, T., Katayama, Y., Yamamoto, T., Hirayama, T.,
and Koyama, S. (1991). Chronic motor cortex stim-
ulation for the treatment of central pain. Acta Neu-
rochirurgica Supplement, 52:137–139.
van Elswijk, G., Maij, F., Schoffelen, J.-M., Overeem, S.,
Stegeman, D. F., and Fries, P. (2010). Corticospinal
beta-band synchronization entails rhythmic gain mod-
ulation. Journal of Neuroscience, 30(12):4481–4488.
Walter, A., Bensch, M., Brugger, D., Rosenstiel, W., Bog-
dan, M., Birbaumer, N., and Gharabaghi, A. (2009).
BCCI - a bidirectional cortical communication inter-
face. In Proceedings of the International Joint Confer-
ence on Computational Intelligence, pages 440–445.
Walter, A., Murguialday, A. R., Sp¨uler, M., Naros, G., Le˜ao,
M. T., Gharabaghi, A., Rosenstiel, W., Birbaumer, N.,
and Bogdan, M. (2012). Coupling BCI and corti-
cal stimulation for brain-state-dependent stimulation:
methods for spectral estimation in the presence of
stimulation after-effects. Frontiers in Neural Circuits,
6:87.
Wassermann, E., Epstein, C., and Ziemann, U. (2008). Ox-
ford Handbook of Transcranial Stimulation (Oxford
Handbooks). Oxford University Press, USA.
Woody, C. D. (1967). Characterization of an adaptive filter
for the analysis of variable latency neuroelectric sig-
nals. Medical & Biological Engineering, 5(6):539–
554.
DynamicsofaStimulation-evokedECoGPotentialDuringStrokeRehabilitation-ACaseStudy
247