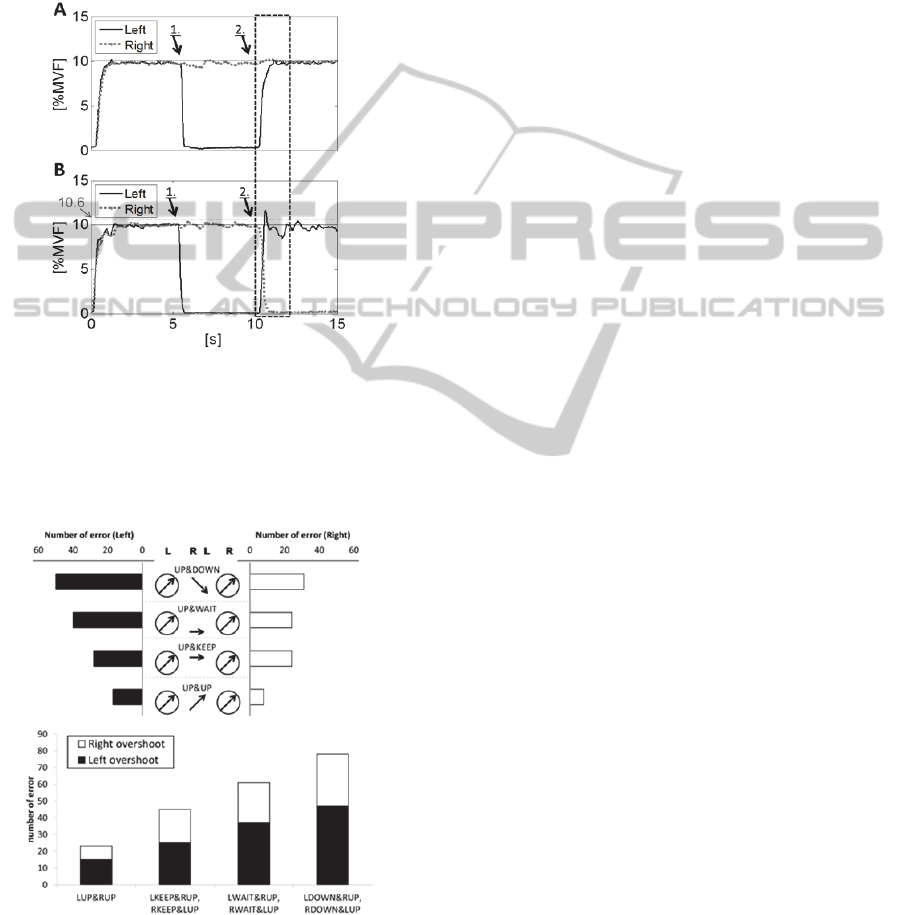
possible. It consists of two switching points;
preliminary 5 s maintain both hands finger pinch
force output at 10%MVF, then disappearing or
appearing the feedback at 5 s (first switching point)
and remained till 10 s, then second switching point
at 10 s after the beginning of the measurement. The
task continues 15 s and participants didn’t know the
timing of switching point (Figure 1).
Figure 1: Representative force outputs for two conditions.
A: Force output of LUP&RKEEP condition. B: Force
output of LUP&RDOWN condition. 1 indicates first
switching point (after 5seconds) and 2 indicates second
switching point (after 10seconds). Rectangle with broken
line indicates analyzing area. Left hand force output
exceeded 10.6%MVF on B, became overshoot error trial.
Figure 2: Number of overshoot error. Graph above shows
a number of left and right overshoot error separately.
Arrows inside the circle denote UP side while arrows in
the middle of them indicate the opposite sides required
force production (UP, DOWN, KEEP and WAIT). Graph
below shows the total number of overshoot error.
2.3 Data Analysis
We focused on second switching point (Figure 1).
There are 4 different force producing types: increase
force output from 0 to 10%MVF (UP), decrease
force output from 10 to 0%MVF (DOWN), keep
maintaining 10%MVF (KEEP) and waiting at
0%MVF force output level (WAIT). Combination of
them with both hands, there are 7 different
conditions: LUP&RUP, LUP&RDOWN,
RUP&LDOWN, LUP&RKEEP, RUP&LKEEP,
LUP&RWAIT and RUP&LWAIT. Participants
engaged three trials on each condition, arranged in
random order. The error trial was defined as when
UP side of the hands’ force output exceeded
10.6%MVF before 2 s from second switching point.
We calculated the error ratio as the number of error
trials per total numbers of trial.
3 RESULTS
Error ratio of each condition was shown in Figure2.
The number of error ratio was the fewest on
UP&UP, total 25/198 (Left: 17/96 Right: 8/96),
followed by KEEP&UP total 52/192 (Left: 28/96,
Right: 24/96) and WAIT&UP total 64/191 (Left:
40/96, Right: 24/95). Most mistakable condition was
DOWN&UP, total 81/192 (Left: 50/96, Right:
31/96). A chi-square test of independence was
performed to examine the relationship between
experimental tasks and overshoot error ratio. The
relation between these variables was
significant,Χ
3, 767
42.3, 0.05.
4 DISCUSSION
When controlling one side of limb’s force output to
certain target, overshoot error is influenced by the
other side’s force producing type. It is easy to
control target force output when both hand’s task are
same force producing type. In contrast, it becomes
difficult to control force output when anti-phase task
is required on the other side. This may because the
attention to the difficulty and complexity of the
other side’s limb movement and/or task disturbs the
control of the force output.
The overshoot error ratio in right hand was lower
than that of left hand. Certainly, the participants
were all right-handed. Dominant limb is specialized
for dynamic, feed-forward controlled unimanual
tasks (Sainburg, 2002). There was relatively lower