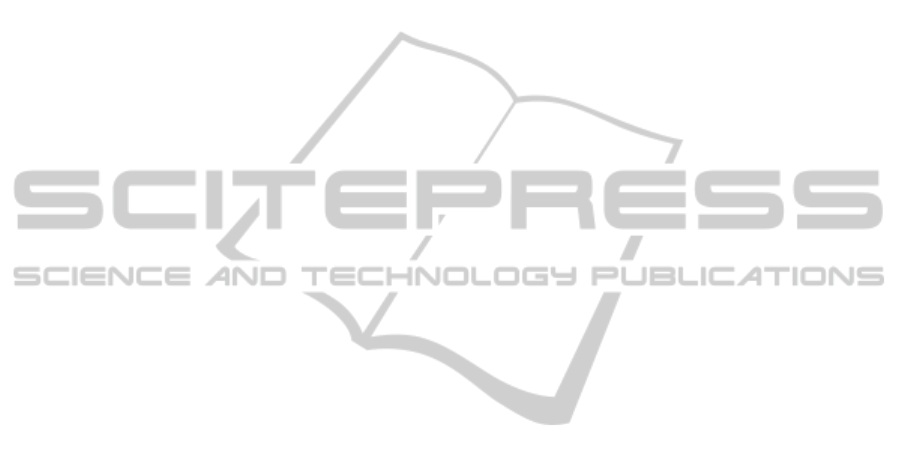
cardiology, aiming at their translation from the
bench to the patient bedside.
REFERENCES
Ametamey, S. M., Honer, M., Schubiger, P. A.: Molecular
imaging with PET, Chem. Rev. 108 (2008) 1501-
1516.
Correia, J. D. G., Paulo, A., Raposinho, P. D., Santos, I.:
Radiometallated peptides for molecular imaging and
targeted therapy Dalton Trans 40 (2011) 6144-6167.
Cutler, C. S., Hennkens ,H. M., Sisay, N., Huclier-Markai,
S., Jurisson, S. S.: Radiometals for Combined Imaging
and Therapy, Chem. Rev. 113 (2013) 858-883.
Hsiao,Y-M., Mathias, C. J., Wey, S-P., Fanwick, P. E.,
Green, M. A.: Synthesis and biodistribution of
lipophilic and monocationic gallium radiopharma-
ceuticals derived from N,N′-bis(3-aminopropyl)-N,N′-
dimethylethylenediamine: potential agents for PET
myocardial imaging with
68
Ga, Nucl. Med. Biol., 36
(2009) 39-45.
Kontos, M. C., Dilsizian,V., Weiland, F., DePuey, G.,
Mahmarian, J. J., Iskandrian, A. E., Bateman, T. M.,
Heller, G. V., Ananthasubramaniam, K., Li, Y.,
Goldman, J. L., Armor, T., Kacena, K. A., LaFrance,
N. D., Garcia, E. V., Babich, J. W., Udelson, J. E.:
Iodofiltic Acid I 123 (BMIPP) Fatty Acid Imaging
Improves Initial Diagnosis in Emergency
DepartmentPatients With Suspected Acute Coronary
Syndromes, J. Am. Coll. Card. 56 (2010) 290-299.
Maria, L., Fernandes ,C., Garcia, R,. Gano, L., Paulo, A.,
Santos, I. C., Santos, I.: Tris(pyrazolyl)methane
99m
Tc
tricarbonyl complexes for myocardial imaging, Dalton
Trans (2009) 603–606.
Morais, G. R., Paulo, A., Santos, I.: Organometallic
Complexes for SPECT Imaging and/or Radionuclide
Therapy, Organometallics 31 (2012a) 5693-5714.
Morais, G. R., Paulo, A., Santos, I.: Synthetic Overview of
Radiolabeled Compounds for β-Amyloid Targeting,
Eur. J. Org. Chem. (2012b) 1279-1293.
Notghi, A., Low, C. S.: Myocardial perfusion
scintigraphy: past, present and future, Brit. J. Radiol.
84 (2011) S229–S236.
Osborn, E. A., Jaffer, F. A.: The Year in Molecular
Imaging, J. Am. Coll. Card. 58 (2012) 317-328.
Paterson, B. M., Donnelly, P. S.: Copper complexes of
bis(thiosemicarbazones): From chemotherapeutics to
diagnostic and therapeutic radiopharmaceuticals.
Chem. Soc. Rev. 40 (2011) 3005–3018.
Strauss, H. W., Narula, J., Orellana, P., Jaimovich, R.,
Stevenson, N., Gonzales, G., Srivastava, S.: Targeting
of vulnerable plaque using [tin-117m]-DOTA-
annexin, J Nucl Med. 54 (Supplement 2) (2013) 1667.
Tamaki, N.; Yoshinaga, K.: Novel iodinated tracers,
MIBG and BMIPP, for nuclear cardiology, J. Nucl.
Cardiol. 18 (2011) 135-143.
Vallabhajosula, S., Nikolopoulou, A.: Radioiodinated
Metaiodobenzylguanidine (MIBG): Radiochemistry,
Biology, and Pharmacology, Semin. Nucl. Med. 41
(2011) 324-33.
Wackers, F. J. T., Berman, D. S., Maddahi, J., Watson, D.
D., Beller, G. A., Strauss, H. W., Boucher, C. A.,
Picard, M., Holman, B. L., Fridrich, ,R., Inglese, E.,
Delaloye, B., Bischofdelaloye, A., Camin, L.,
Mckusick K.: Technetium-99m Hexakis 2-
Methoxyisobutyl Isonitrile: Human Biodistribution,
Dosimetry, Safety, and Preliminary Comparison to
Thallium-201 for Myocardial Perfusion Imaging. J
Nucl Med 30 (1989) 301-311.
Yu, M., Nekolla, S. G., Schwaiger, M., Robinson, S. P.:
The next generation of cardiac positron emission
tomography imaging agents: Discovery of Flurpiridaz
F-18 for detection of coronary disease, Semin. Nucl.
Med. 41 (2011) 305-313.
CARDIOTECHNIX2013-InternationalCongressonCardiovascularTechnologies
78