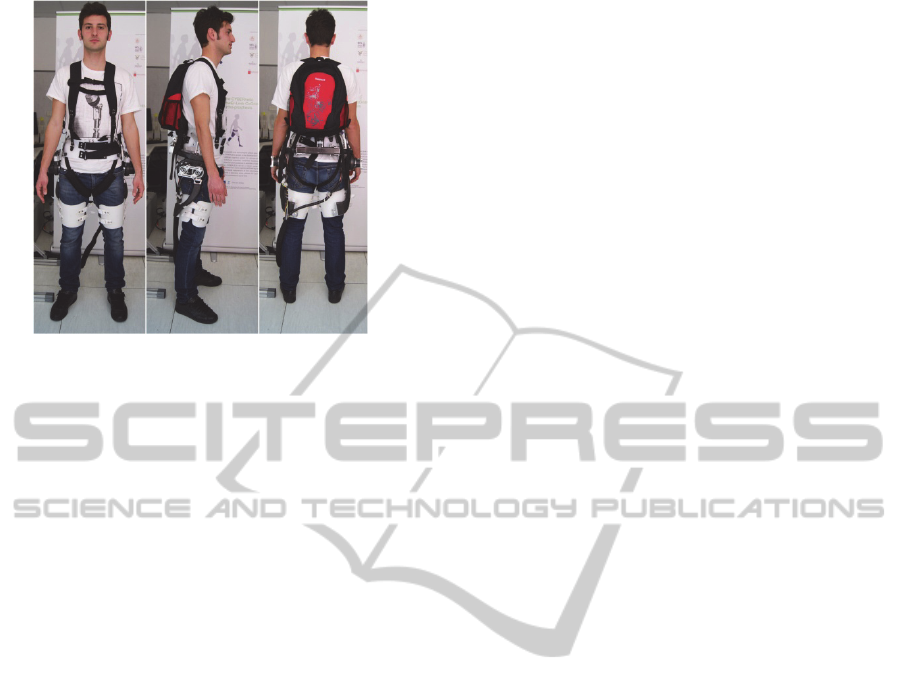
Figure 4: A subject wearing the exoskeleton.
4 DISCUSSION
Recorded data and feedback from subjects (Figure 4)
that tested the device showed promising
performance for the developed exoskeleton and
encourage to progress with a more extensive
experimental characterization. The target users for
the presented exoskeleton will be elderly people that
need assistance to recover a more stable gait pattern;
in addiction the device is addressed to reduce the
metabolic consumption in lower-limb transfemoral
amputees providing them with assistance during
walking and other tasks. In general several kinds of
patients affected by gait disorders could take
advantages from the presented device.
ACKNOWLEDGEMENTS
This work was supported in part by the EU within
the CYBERLEGs project (FP7-ICT-2011-2.1 Grant
Agreement #287894) and by Fondazione Pisa within
the IUVO project (prog. 154/11).
REFERENCES
Banala, S. K., Agrawal, S. K., Fattah, A. et al., 2006.
Gravity-Balancing Leg Orthosis and Its Performance
Evaluation, IEEE Transactions on Robotics,
22(6):1228-1239.
Beyl, P., Van Damme, M., Van Ham, R., et al., 2010.
Design and control of a lower limb exoskeleton for
robot-assisted gait training. Applied Bionics and
Biomechanics, 6(2):229 – 243.
Burge, R., Dawson-Hughes, B., Solomon, D. H., et al.,
2007. Incidence and Economic Burden of
Osteoporosis-Related Fractures in the United States,
2005–2025. Journal of Bone and Mineral Research,
22: 465–475.
Cempini, M., Giovacchini, F., Vitiello, N., et al., 2013.
NEUROExos: A Powered Elbow Orthosis for Post-
Stroke Early Neurorehabilitation. In Proceedings of
35th Annual International Conference of the IEEE
EMBS, 342-345.
Dollar, A., Herr, H., 2008. Lower extremity exoskeletons
and active orthoses: challenges and state-of-the-art.
IEEE Transactions on Robotics, 24(1):144 158.
Kazerooni, H., Steger, R., 2006. The Berkeley Lower
Extremity Exoskeleton. Journal of Dynamic Systems,
Measurement and Control, 128(1):14-25.
Pons, J. L, 2010. Rehabilitation Exoskeletal Robotics.
IEEE Engineering in Medicine and Biology Magazine,
29(3): 57-63.
Pratt, G., Williamson, M. M., 1995. Series elastic
actuators. In Proc. IEEE Int. Conf. Intell. Robots Syst.,
Pittsburgh, PA, 339–406.
Ronsse, R., Vitiello, N., Lenzi, T., et al., 2011. Human-
robot synchrony: flexible assistance using adaptive
oscillators, IEEE Transactions on Biomedical
Engineering, 58(4):1001-1012.
Ronsse, R., Lenzi, T., Vitiello, N., et al., 2011. Oscillator-
based assistance of cyclical movements: model-based
and model-free approaches, Medical and Biological
Engineering and Computing, 49(10):1173-1185.
Sawicki, G., Gordon, K., Ferris, D., 2005. Powered lower
limb orthoses: applications in motor adaptation and
rehabilitation. In 9th International Conference on
Rehabilitation Robotics, 206-211.
Snijders, A. H., van den Warrenburg, B.P., Giladi, N., et
al., 2007. Neurological gait disorders in elderly
people: clinical approach and classification. Lancet
Neurol., 6(1):63-74.
Stolze, H., Klebe, S., Baecker, C., 2005. Prevalence of gait
disorders in hospitalized neurological patients. Mov
Disord, 20(1): 89–94.
Sulzer, J., Roiz, R., Peshkin, M., et al., 2009. A Highly
Backdrivable, Lightweight Knee Actuator for
Investigating Gait in Stroke. IEEE Transactions on
Robotics, 25(3):539-548.
Verghese, J., Levalley, A., Hall, C. B., et al., 2006.
Epidemiology of gait disorders in community-residing
older adults. J Am Geriatr Soc, 54: 255–261.
Walsh, C. J., Endo, K., Herr, H., 2007. A quasi-passive
Legacy Exoskeleton for load-carrying augmentation.
International Journal of Humanoid Robotics,
4(3):487-506.
Weinberg, B., Nikitczuk, J., Patel, S., et al., 2007. Design,
Control and Human Testing of an Active Knee
Rehabilitation Orthotic Device. In Proceedings of
IEEE International Conference on Robotics and
Automation, 4126-4133.
Wilson, R. S., Schneider, J. A., Beckett, L. A., et al., 2002.
Progression of gait disorder and rigidity and risk of
death in older persons. Neurology, 58: 1815–1819.
Winter D. A., 2009. Biomechanics and Motor Control of
ALight-weightExoskeletonforHipFlexion-extensionAssistance
197