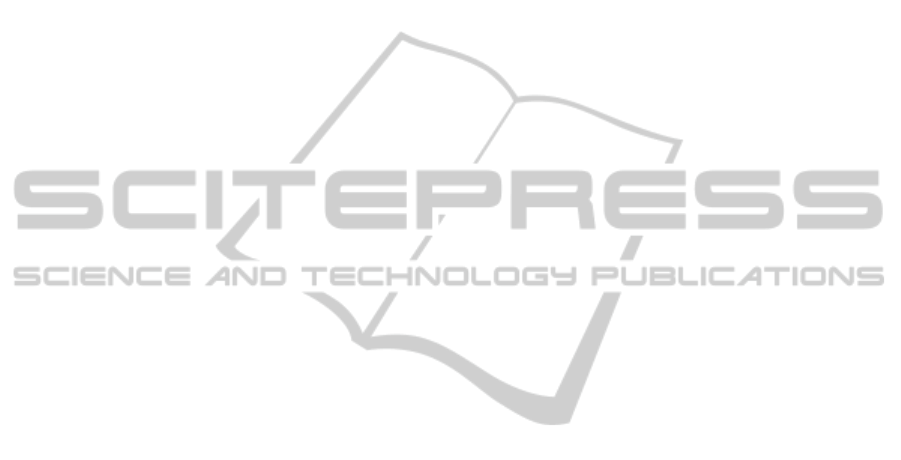
ity, as well as an ever increasing use photoplethysmo-
graphic monitors in clinical practice create conditions
that promote implementation of the proposed method
for screening diagnosis of PAOD.
Restrictions imposed in connection with the use
of PPG are associated with weak signals generated by
motion artifacts or with the limited peripheral blood
flow (F. Javed et al., 2010). The numerical analysis
of the PPG signals proposed in this paper can be ap-
plied to pulse waveforms obtained by other registra-
tion techniques.
The results obtained in the study of LF waves as-
sociated with sympathetic nervous system are also
presented. The spectral analysis of the PPG signal
shows, that LF pulsations of blood flow of subjects
with PAOD are higher then of healthy ones. The
cause of it can be microcirculation adaptation to the
decreased blood flow in the limb. The amplitude of
LF oscillations becomes smaller after surgery. This
can be explained by suppressing active mechanisms,
induced by peripheral nervous fibers trauma.
ACKNOWLEDGEMENTS
The work is undertaken under financial support of
RFBR-Ural 11-01-96018.
REFERENCES
Allen, J. (2007). Photoplethysmography and its application
in clinical physiological measurement. Physiological
Measurement, 28(3):R1.
Allen, J., Overbeck, K., Nath, A. F., Murray, A., and
Stansby, G. (2008). A prospective comparison
of bilateral photoplethysmography versus the ankle-
brachial pressure index for detecting and quantifying
lower limb peripheral arterial disease. Journal of Vas-
cular Surgery, 47(4):794 – 802.
Bernjak, A. and Stefanovska, A. (2007). Importance of
wavelet analysis in laser doppler flowmetry time se-
ries. In Engineering in Medicine and Biology Society,
2007. EMBS 2007. 29th Annual International Confer-
ence of the IEEE, pages 4064–4067.
Carter, S. A. (1968). Indirect systolic pressures and pulse
waves in arterial occlusive disease of the lower ex-
tremities. Circulation, 37(4):624–637.
Erts, R., Spigulis, J., Kukulis, I., and Ozols, M. (2005). Bi-
lateral photoplethysmography studies of the leg arte-
rial stenosis. Physiological Measurement, 26(5):865.
F. Javed, P. M. Middleton, P. Malouf, G.S H Chan, A.V.
Savkin, N.H. Lovell, E. Steel, and J. Mackie (2010).
Frequency spectrum analysis of finger photoplethys-
mographic waveform variability during haemodialy-
sis. Physiological Measurement, 31(9):1203.
Goupillaud, P., Grossmann, A., and Morlet, J. (1984).
Cycle-octave and related transforms in seismic signal
analysis. Geoexploration, 23(1):85–102.
Holohan, T. (1996). Plethysmography: safety, effectiveness,
and clinical utility in diagnosing vascular disease. Di-
ane Publishing Company.
Leondes, C. (2002). Computational Methods in Biophysics,
Biomaterials, Biotechnology and Medical Systems:
Algorithm Development, Mathematical Analysis and
DiagnosticsVolume I: Algorithm TechniquesVolume
II: Computational MethodsVolume III: Mathemati-
cal Analysis MethodsVolume IV: Diagnostic Methods.
Springer.
Lin, C.-H. (2011). Assessment of bilateral photoplethys-
mography for lower limb peripheral vascular occlu-
sive disease using color relation analysis classifier.
Computer Methods and Programs in Biomedicine,
103(3):121 – 131.
Nesme-Ribes E., Frick P., Sokoloff D., Zakharov V., Ribes
J.-C., Vigouroux A., and Laclare F. (1995). Wavelet
analysis of the Maunder minimum as recorded in solar
diameter data. Academie des Sciences Paris Comptes
Rendus Serie B Sciences Physiques, 321:525–532.
Nichols, W. W., O’Rourke, M. F., and Vlachopoulos, C.
(2011). McDonald’s Blood Flow in Arteries, 6th
ed: Theoretical, Experimental and Clinical Princi-
ples. Hodder Arnold Publishers, 6 edition.
Nitzan, M., Babchenko, A., Khanokh, B., and Landau, D.
(1998). The variability of the photoplethysmographic
signal - a potential method for the evaluation of the au-
tonomic nervous system. Physiological Measurement,
19(1):93.
Norgren, L., Hiatt, W., Dormandy, J., Nehler, M., Har-
ris, K., and Fowkes, F. (2007). Inter-society con-
sensus for the management of peripheral arterial dis-
ease (tasc ii). Journal of Vascular Surgery, 45(1,
Supplement):S5 – S67. ¡ce:title¿TASC II¡/ce:title¿
¡ce:subtitle¿Inter-Society Consensus for the Manage-
ment of PAD¡/ce:subtitle¿.
O’Rourke, M. F. and Kelly, R. P. (1993). Wave reflection
in the systemic circulation and its implications in ven-
tricular function. Journal of hypertension, 11(4):327–
337.
Schuhfried, O., Wiesinger, G., Kollmitzer, J., Mittermaier,
C., and Quittan, M. (2003). Fourier analysis of
impedance rheography for peripheral arterial occlu-
sive disease. European Journal of Applied Physiology,
89(3):384–386.
Sherebrin, M. and Sherebrin, R. Z. (1990). Frequency anal-
ysis of the peripheral pulse wave detected in the finger
with a photoplethysmograph. Biomedical Engineer-
ing, IEEE Transactions on, 37(3):313–317.
BIOSIGNALS2014-InternationalConferenceonBio-inspiredSystemsandSignalProcessing
154