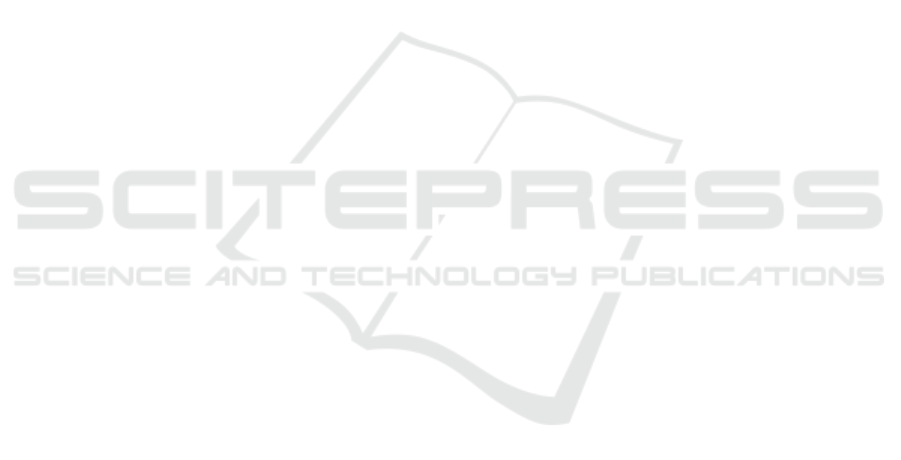
Noninvasive Glucose Monitoring by Mid-infrared
Self-emission Method
Yen-Chun Yeh, Sheng Yang, Fan Zhao and Dominik Schmidt
Department of Electrical Engineering, International Technological University, San Jose, CA, U.S.A.
Keywords: Non-invasive Glucose Monitoring, Mid-infrared Self-emission, Skin Glucose, Blood Glucose.
Abstract: In this article we present a non-invasive glucose monitoring technique by measuring human body mid-
infrared self-emission. The human body is a black body radiator that provides a stable temperature and
infrared radiation; thus the human body is considered a continuous radiation energy source in the mid-
infrared range. The fingerprint spectrum of glucose shows strong peaks between 8.5 m to 10.4 m,
therefore, measuring the self-emission form human body in the mid-infrared range allows estimation of
glucose concentration. Using a simple and miniaturizable design with a tunable Fabry-Perot filter (FPF) and
a thermal detector, glucose concentration can be measured through the human skin.
1 INTRODUCTION
It has been decades since blood glucose monitoring
techniques were introduced for diabetes patients to
help them with daily management of diabetes
treatments, including diet control, oral medication
and insulin injection. The traditional measurement of
blood glucose concentration requires the patients to
stab themselves in the finger with a needle to extract
the blood to the skin surface so that the blood can be
collected for enzymatic reaction and analysis.
However, in the past decade, the desire to avoid the
pain resulting from the puncture and to realize
continuous blood glucose monitoring has driven
research in a variety of non-invasive glucose sensing
techniques. The non-invasive glucose monitoring
techniques can be classified into two different types,
transdermal (Rao, 1993; Volden, 1980; Gebhardt,
2001) and optical (Shen, 2003; Nelson, 2006;
Enejder, 2005). The main idea of transdermal
glucose sensing techniques is to extract the glucose
from the interstitial fluid to the outer surface of the
skin, where the glucose will be collected and
analyzed by a traditional glucose sensor. The
popular transdermal techniques include reverse
iontophoresis, sonophoresis and skin suction blister
technique (Kost, 2000.). Essentially, the key point of
these methods lies in the different approaches to
collect the glucose. The technique used to identify
and quantify glucose remains the same, which is
based on enzymes. By contrast, the optical methods
aim at identifying the unique spectral signature of
glucose and exploring the best way to calibrate and
quantify optical measurements. Due to the noise
coming from the skin, both SNR and sensitivity are
affected. A significant advantage of this system is
not requiring an enzyme replacement.
Non-invasive optical glucose monitoring
methods basically include three different techniques
to measure glucose in the infrared region. Of the
three methods, near-infrared (NIR) (Nelson, 2006)
spectroscopy, mid-infrared (MIR) (Shen, 2003)
spectroscopy, Raman spectroscopy (Enejder, 2005),
only MIR spectroscopy can measure glucose without
a light source. Because the human body is an
excellent black body, it will cause heat emission in
the MIR wavelength range. Therefore, self-emission
from the human body can generate good target
glucose spectra, and enable an easy to measure
glucose concentration in the human body. In the
mid-IR, glucose has a stronger absorption than most
other chemicals, and if avoids most of the water
signal peaks. Also, using the MIR method can avoid
the use of high-energy light sources on the skin,
which can cause burns. Furthermore, these
advantages facilitate the implementation of the non-
invasive system on a single microelectromechanical
system (MEMS) chip, which is our long-term goal.
Therefore, we chose to use thermal emission
spectroscopy as our scheme to realize a non-invasive
glucose sensor. The most significant advantage is
107
Yeh Y., Yang S., Zhao F. and Schmidt D..
Noninvasive Glucose Monitoring by Mid-infrared Self-emission Method.
DOI: 10.5220/0004750101070111
In Proceedings of the International Conference on Biomedical Electronics and Devices (BIODEVICES-2014), pages 107-111
ISBN: 978-989-758-013-0
Copyright
c
2014 SCITEPRESS (Science and Technology Publications, Lda.)