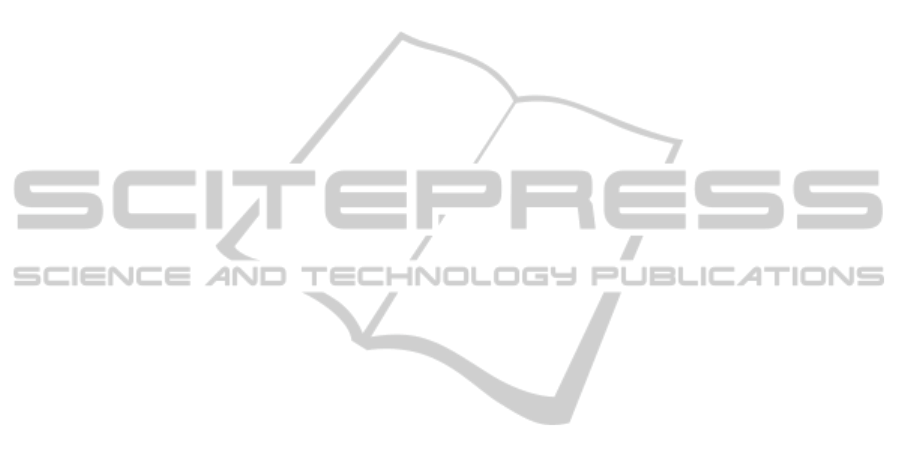
´operational rules´. Students do not report on
theoretical understanding or models they (re-
)constructed.
Concerning the second research question, it is
found that the games screen lay-out has a strong
though undesired impact on students’ mental model
of electrical circuits and student learning. The way
things are presented apparently strongly influences
the students´ way of ‘looking at things’. This
‘topological mimicking’ seems to be a very powerful
learning mechanism. The games lay-out, and
probably its structure as well, sends out a very
strong message about ‘how things are’. Though not
entirely effective in the case of our project, the
strength of this mechanism is potentially valuable
for designing serious games.
Concerning the use of serious games in
classroom, it became clear that ‘open inquiry’ alone
is not the way to go. An approach balancing gaming
and deep cognitive processing in alternating phases
seems much more fruitful. In this a rule of thumb
helping teacher in a practical way could be: “treat
serious games as experiments or practical exercises”.
Our research has been on a fairly small scale, and
as such its outcomes may be easily over generalized.
But they point toward two particular issues of
importance for larger scale future research:
- developing a pedagogical approaches balancing
between play and open inquiry on one hand and
stimulating and structuring deep cognitive
processing on the other. Put more generally:
seeking a productive balance between flow and
reflection.
- taking advantage of the strong modelling impact
the lay-out and the structure of the game have on
student learning.
ACKNOWLEDGEMENTS
This research was funded by the SLOA fund of the
Dutch ‘onderwijs cooperation’ seeking to promote
secondary school teachers doing educational
research in cooperation with academic educational
researchers.
REFERENCES
Anderson, B. (1986). The experiential gestalt of causation:
a common core to pupils’ preconceptions in science.
European journal of science education, 8, 155-171.
Csikszentmihaly, M. (2009). Creativity: Flow and the
Psychology of Discovery and Invention.
HarperCollins, New York.
Csikszentmihalyi, M.(2000). Das Flow-Erlebnis. Jenseits
von Angst und Langeweile: im Tun aufgehen [Beyond
boredom and anxiety] (8th. Ed.).Stuttgart, Germany:
Klett-Cotta.
Dekker, J., Jacobs, A., van Hensberg, V. (2010). Informal
user test of E&E electrical endeavours. Internal report.
Dreyfus, S., E., & Dreyfus, H. L. (February 1980). A Five-
Stage Model of the Mental Activities Involved. In
Directed Skill Acquisition. Washington, DC: Storming
Media. Retrieved June 13, 2010.
http://www.dtic.mil/cgi-bin/GetTRDoc?AD=ADA08
4551&Location=U2&doc=GetTRDoc.pdf.
Duit, R. (2009). Bibliography STCSE: Students’ and
teachers’ conceptions and science education.
http://www.ipn.uni-kiel.de/aktuell/stcse/stcse. html.
Accessed 20 April 2009.
Duit, R., & Schecker, H. (2007). Teaching Physics. In S.
K. Abell & N. G. Lederman (Eds.), Handbook of
research on science education (pp. 599–629). New
York: Routledge.
Duit, R., & Von Rhoeneck, C. (1998). Learning and
understanding key concepts of electricity. In
A.Tiberghien, E. Leonard Jossem, & J. Barojas (Eds.),
Connecting research in physics education with teacher
education. International Commission on Physics
Education.
Engelhardt, P. V., & Beichner, R. J. (2004). Students’
understanding of direct current resistive electrical
circuits. American Journal of Physics, 72(1), 98–115.
Gravemeijer, K., & Cobb, P. (2006). Design Research
from a Learning Design Perspective. In J. Van den
Akker, K. Gravemeijer, S. McKenney & N. Nieveen
(Eds.), Educational design research. London:
Routledge.
Hart, C. (2008). Models in physics, models for physics
learning, and why the distinction may matter in the
case of electric circuits. Research in Science
Education, 38, 529–544.
Jaakkola, T., Nurmi, S., & Veermans, K. (2010). A
comparison of students' conceptual understanding of
electric circuits in simulation only and simulation-
laboratory contexts. Journal of research in science
teaching. http://onlinelibrary.wiley.com/doi/10.
1002/tea.20386/pdf.
Kirschner, P. A., Sweller, J., & Clark, R. E. (2006). Why
Minimal Guidance During Instruction Does Not
Work: An Analysis of the Failure of Constructivist,
Discovery, Problem-Based, Experiential, and Inquiry-
Based Teaching. Educational Psychologist, 41(2), 75-
86. http://igitur-archive.library.uu.nl/fss/2006-1214 -
211848/kirschner_06_minimal _guidance.pdf.
Kock, Z. D. Q. P., Taconis, R., Bolhuis, S. M &
Gravemeijer, K.P.E. (2013). Some key Issues in
creating inquiry-based instructional practices that aim
at the understanding of simple electric circuits.
Research in Science Education, 43(2), 579-597.
Kolb, D. (1984). Experiential learning: Experience as the
source of learning and development. New Jersey:
Prentice Hall.
CSEDU2014-6thInternationalConferenceonComputerSupportedEducation
12