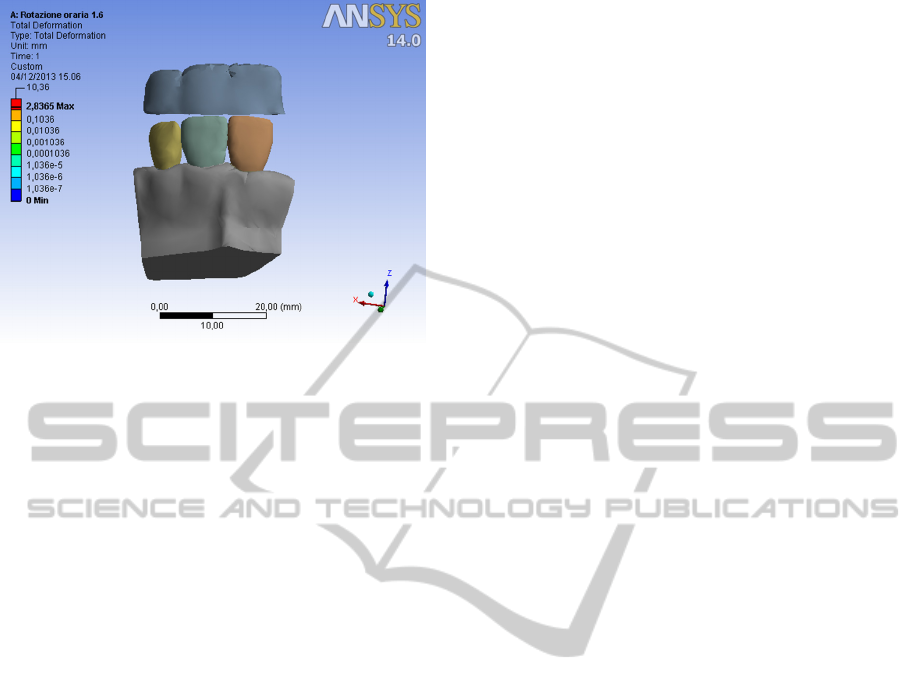
Figure 10: Aligner over the teeth at the starting point of
the simulation.
5.3 Model Validation
After obtaining the desired results from the
simulations will be performed an experimental
validation comparing them with that obtained by
other techniques. Some ideas are:
5.3.1 Photoelasticity
Photoelasticity is a full-field technique which
directly provides the information of principal stress
difference and the orientation of principal stress
direction by fringe analysis of components made of
birifrangent materials. The thermoplastic material
which composes the aligner is transparent and is
characterized by having photoelastic properties. The
introduction of customized photoelastic analyses for
real components would greatly enhance the
detection of possible criticalities arising from a
challenging application such as the optimisation of
removable aligners.
5.3.2 Electronic Measurement Device
Some researchers have developed electronic
systems, based on strain gauges, able to measure
forces and moments during a simulation of an
orthodontic treatment. The comparison between the
FEM model with the measurements obtained by the
electronic device can give an idea about the
accuracy of the model. (Hahn et al., 2010)
5.3.3 Clinical Tests on Real Patients
The best way to validate the model would rely on
the comparison of the numerical results with those
obtained by clinical tests involving real orthodontic
treatments.
6 EXPECTED OUTCOME
The present research project is focused on the study
of how the orthodontic aligners work and on the
optimization of their design process. The appropriate
definition of parameters as shape, dimensions of
attachments, thickness and material of the aligner
would allow the definition of a more predictable
treatment. Moreover, shorter treatment times would
be characterized by less discomfort for the patient
and lower costs since performed by a lower number
of aligners.
The results obtained by this research could be
also used to extend the use of invisible aligners to
malocclusion problems which are presently treated
by different orthodontic appliances, and to improve
their production process.
REFERENCES
Barbagallo, L., Shen, G., Jones, A., Swain, M., Petocz, P.,
& Darendeliler, M. (2008). A novel pressure film
approach for determining the force imparted by clear
removable thermoplastic appliances. Annals of
Biomedical Engineering , 335-341.
Barone, S., Paoli, A., & Razionale, A. (2013b). Computer-
aided modelling of three-dimensional maxillofacial
tissues through multi-modal imaging. Proceedings of
the Institution of Mechanical Engineers, PArt H:
Journal of Engineering in Medicine , 227,89-104.
Barone, S., Paoli, A., & Razionale, A. (2013a). Creation of
3D Multi-Body Orthodontic Models by Using
Independent Imaging Sensors. Sensors , 13,2033-
2050.
Beers, A., Choi, W., & Pavlovskaia, E. (2003). Computer-
assisted treatment planning and analysis. Orthodontics
& craniofacial research , 117-125.
Field, C., Ichim, I., Swain, M., Chan, E., Darendeliler, M.,
Li, W., et al. (2009). Mechanical responses to
orthodontic loading: A 3-dimensional finite element
multi-tooth model. American Journal of Orthodontics
and Dentofacial Orthopedics , 174-181.
Fill, T. S., Toogood, R. W., Major, P. W., & Carey, J. P.
(2012). Analytically determined mechanical properties
of, and models for the. Journal of Biomechanics , 9-
16.
Hahn, W., Engelke, B., Jung, K., Dathe, H., Fialka-Fricke,
J., Kubein-Meesenburg, D., et al. (2010). Initial forces
and moments delivered by removable thermoplastic
appliances during rotation of an upper central incisor.
Angle Orthodontist , 80,2,239-246.
BIOSTEC2014-DoctoralConsortium
34