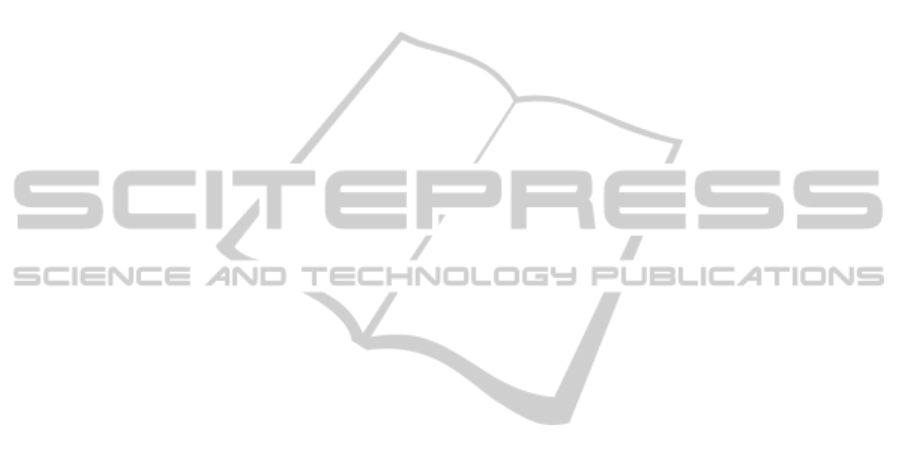
mental state). Heart rate variability (HRV) is the
physiological phenomenon in which the heart rate
oscillates around its mean value due to external
influences from the autonomic nervous system
(ANS), or other mental and physical factors (Malik,
M., 1990; Malik, M., 2006). It’s a marker to study
the activity of the regulatory mechanisms and to
analyse the effects (excitatory and inhibitory) they
have upon the heart rate.
HRV can be analysed on time or frequency
domains. Through the analysis of the root mean
square of the
successive RR intervals we can obtain a
measure of the sympathetic and parasympathetic vagal
activity (Clifford, G., 2002). One way to measure the HR
is through the Blood Volume Pulse (BVP) sensor. With
the cardiac pulse, erythrocytes will change the spatial
alignment and this will affect the blood’s opacity. The
BVP sensor makes use of opacity variations from the tip
of the finger to gather the cardiac pulse on a certain
instant.This study
aims to analyse the effects of
electrical stimulation on human physiology, based
on skin’s galvanic response and on Blood Volume
Pulse (BVP) signals.
2 METHODS
2.1 Subjects and Room Conditions
In this study a total of 10 healthy subjects, composed
of 5 males and 5 females, with a mean age of 24.10
years (standard deviation of 2.38 years), were
submitted to a previously developed heart rate and
skin conductance acquisition protocol. All of the
subjects were healthy, with no physical or
neurophysiological disorders registered.
The subjects were seated on a comfortable chair
with both their arms relaxed. It was required of them
to feel comfortable for the acquisition to begin.
Earmuffs were placed on the subjects’ ears to
prevent any noise distraction. Moreover the room
was kept silent during the acquisition and the
subjects were asked to remain motionless and
relaxed.
The stimuli were applied through two disposable
electrodes on the median nerve of the left wrist and
two electrodermal activity acquisition electrodes
were placed on the abductor pollicis muscle and on
the 3
rd
palmar interosseous muscle of the right hand.
An oximeter was also placed on the index finger of
the right hand to measure the heart rate of the
subjects. The sensor makes use of colour variations
from the tip of the index finger (due to blood’s
opacity) to gather the blood volume on a certain
instance in time.
Although the majority of the subjects didn’t
know about the acquisition protocol, the acquisition
had to be repeated on two of the subjects (1 and 2)
due to low quality signal reading.
2.2 Acquisition Protocol
The first part of the acquisition took 4 minutes and
no stimuli were applied to the subjects. This segment
of the acquisition protocol had the purpose of
acquiring the basal BVP and EDA.
The second segment featured the application of
the electrical stimuli on the medial nerve of the left
wrist. Square current modulated waveform was
used. The electrical stimuli consisted of 6 bursts of
20 repetitive stimuli each and with increasing
intensities (ranging from 5 to 30 mA). In each burst
the interval between stimuli was 0.9 seconds and the
time interval between bursts was of 10 seconds.
The final segment of the acquisition was similar
to the first one, a 4-minute acquisition to record the
subjects’ recovery after the application of stimuli.
Through all of the phases, these segments were
recorded at a 2000Hz acquisition rate. A combined
wireless, miniaturized and synchronized unit was
specifically developed for multi-biosignal
acquisition (Plux, 2014) and nerve stimulation
(Araújo, T., 2012).
2.3 Processing
After the acquisition from all subjects the data was
processed using a script in Python. The data was
stored in a .txt file (for each subject) and organized
in columns, each column belonging to a certain
parameter and each line to a recorded frame.
The data recorded was processed according to
steps bellow:
1. Conversion of frames to seconds, since 1000
frames corresponded to a single second.
2. Search for all HR peaks. HR data was plotted
in a time/HR graph to ensure all peaks found by the
script matched the plotted peaks.
3. Determination of mean heart rate for all 3
segments.
4. Determination of HR amplitude and HRV for
all 3 segments.
5. Analysis of EDA time latency, response rise
time and readaption slope via plotting and linear
regression.
These steps were repeated for all 10 subjects.
BIOSIGNALS2015-InternationalConferenceonBio-inspiredSystemsandSignalProcessing
236