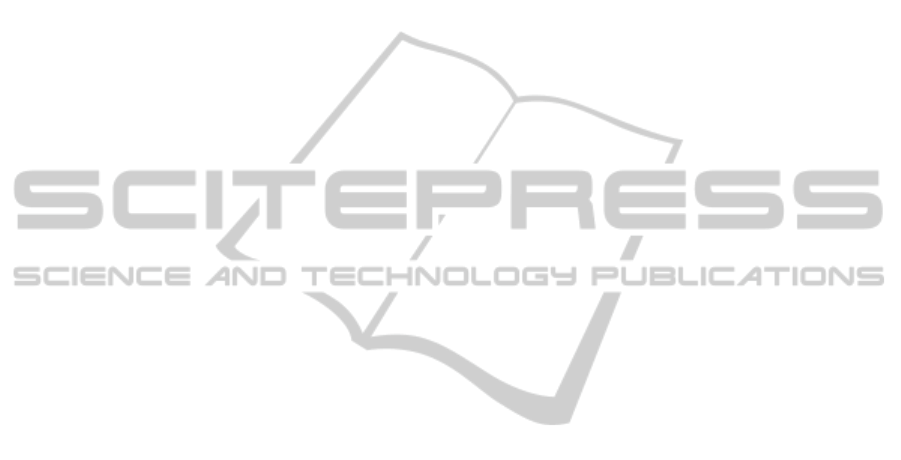
3-6 May, pp. 660-661.
Baard, E., 2001. People Power: Capturing The Body’s
Energy For Work On and Off Earth. (Online)
Available at: http://extropians.weidai.com/extropians.
4Q01/3513.html (Accessed September 2014).
Barroca, N. et al., 2012. Electromagnetic Energy
Harvesting for Wireless Body Area Networks with
Cognitive Radio Capabilities. Proceedings of URSI.
Biagioni, E. & Bridges, K., 2002. The Application of
Remote Sensor Technology to Assist the Recovery of
Rare and Endangered Species. Special issue on
Distributed Sensor Networks for the International
Journal of High Performance Computing
Applications, Volume 16, pp. 315-324.
Biagioni, E. & Chen, S., 2004. A Reliability Layer for Ad-
Hoc Wireless Sensor Network Routing. Proceedings
of the 37th Annual Hawaii International Conference
on System Sciences.
Bregar, K. & Avbelj, V., 2013. Multi-Functional Wireless
Body Sensor - Analysis of Autonomy. Proceedings of
MEET & GVS on the 36th International Convention
MIPRO 2013, pp. 346-349.
Chaboyer, W. et al., 2008. Predictors of Adverse Events in
Patients After Discharge From the Intensive Care Unit.
Am. J. Crit. Care, Volume 17, pp. 255-263.
Coronel, P. et al., 2004. Wireless body area and sensor
networks. Wireless World Research Forum, Briefings.
Cotton, S. L. & Scanlon, W. G., 2007. Characterization
and modeling of the indoor radio channel at 868 MHz
for a mobile bodyworn wireless personal area network.
IEEE Antennas and Wireless Propagation Letters,
Volume 6, pp. 51-55.
Drude, S., 2007. Requirements and Application Scenarios
for Body Area Networks. Mobile and Wireless
Communications Summit, 16th IST.
Dzenowagis, J. & Kernen, G., 2005. Connecting for
health: global vision, local insight: Report for the
World Summit on the Information Society, Geneva:
World Health Organization.
Ekeland, A., Bowes, A. & Flottorp, S., 2010.
Effectiveness of telemedicine: A systematic review of
reviews. Int. J. Med. Inform, Volume 79, pp. 736-771.
European Union, 2004. eHealth Ministerial Declaration,
22 May 2003. In: E-Health: Current Situation and
Examples of Implemented and Beneficial E-Health
Applications. Brussels: IOS Press, pp. 35-38.
Fortuna, C. & Mohorčič, M., 2009. Trends in the
development of communication networks: Cognitive
networks. Computer Networks, Volume 53, pp. 1354-
1376.
Fortuna, C., Mohorčič, M. & Filipič, B., 2008.
Multiobjective Optimization of Service Delivery Over
a Heterogeneous Wireless Access System.
Proceedings of the IEEE International Symposium on
Wireless Communication Systems, ISWCS 2008, pp.
133-137.
Golmie, N., Cypher, D. & Rebala, O., 2005. Performance
analysis of low rate wireless technologies for medical
applications. Computer Communications, Volume 28,
pp. 1266-1275.
Ikehara, C. S., Biagioni, E. & Crosby, M. E., 2007. Ad-
Hoc Wireless Body Area Network for Augmented
Cognition Sensors. In: D. Schmorrow & L. Reeves,
eds. Augmented Cognition. Berlin, Heidelberg:
Springer-Verlag, p. 38–46.
Jingling, F., Wei, L. & Yang, L., 2010. Performance
Enhancement of Wireless Body Area Network System
Combined with Cognitive Radio. Proceedings of the
International Conference on Communications and
Mobile Computing, CMC 2010, pp. 313-317.
Pesko, M. et al., 2014. Smartphone with augmented
gateway functionality as opportunistic WSN gateway
device. Wireless Personal Communications, Volume
78, pp. 1811-1826.
Rohde, J. & Toftegaard, T. S., 2011. Adapting Cognitive
Radio Technology for Low-Power Wireless Personal
Area Network Devices. Wireless Pers. Commun.,
Volume 58, pp. 111-123.
Steenkiste, P., Sicker, D., Minden, G. & Raychaudhuri,
D., 2009. Future Directions in Cognitive Radio
Network Research. NSF Workshop Report.
Tomašić, I., Frljak, S. & Trobec, R., 2013. Estimating the
universal positions of wireless body electrodes for
measuring cardiac electrical activity. IEEE Trans. Inf
.Technol. Biomed., Volume 60, pp. 3368-3374.
Trobec, R., Depolli, M. & Avbelj, V., 2010. Wireless
network of bipolar body electrodes. Proceedings of the
7th International Conference on Wireless On-demand
Network Systems and Services, WONS 2010, p. 145–
149.
Trobec, R., Rashkovska, A. & Avbelj, V., 2012. Two
proximal skin electrodes – a body sensor for
respiration rate. Sensors, Volume 12, pp. 13813-
13828.
Trobec, R. & Tomašić, I., 2011. Synthesis of the 12-lead
electrocardiogram from differential leads. IEEE Trans.
Inf .Technol. Biomed., Volume 15, pp. 615-621.
World Health Organization, 2006. eHealth tools and
services: Needs of the Member States. Report of the
Global Observatory for eHealth.
CoexistenceofWirelessSystemsforRemoteMonitoringofVitalFunctionsintheUnlicensedISMBand
633