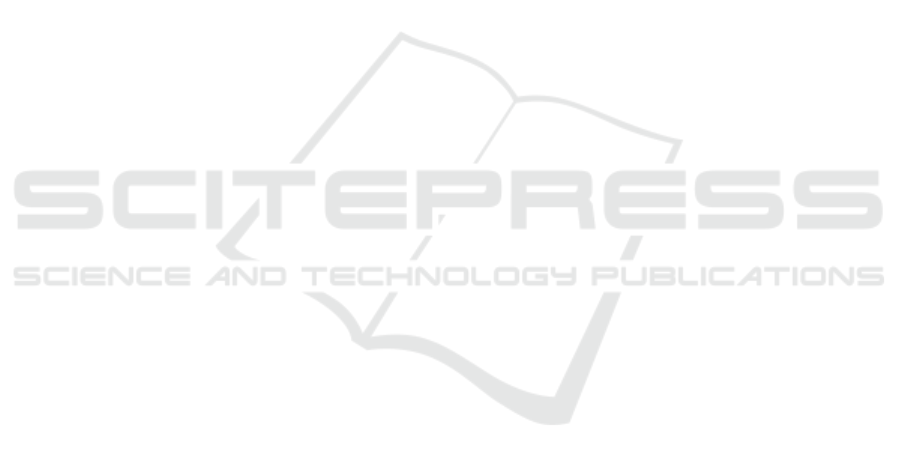
Castelnuovo, G., Priore, C. L., Liccione, D., and Cioffi, G.
(2003). Virtual reality based tools for the rehabilita-
tion of cognitive and executive functions: the v-store.
Psychology, pages 311–326,
Choi, I. and Ricci, C. (1997). Foot-mounted gesture de-
tection and its application in virtual environments. In
Proc. IEEE Int. conf. on Systems, Man and Cybernet-
ics, volume 5, pages 4248–53. IEEE Computer Soci-
ety.
Cipresso, P., Gaggioli, A., Serino, S., Pallavicini, F.,
Raspelli, S., Grassi, A., and Riva, G. (2012). Eeg
alpha asymmetry in virtual environments for the as-
sessment of stress-related disorders. Studies In Health
Technology And Informatics, 173:102–104.
Cipresso, P., Paglia, F. L., Cascia, C. L., Riva, G., Al-
bani, G., and Barbera, D. L. (2013). Break in voli-
tion: A virtual reality study in patients with obsessive-
compulsive disorder. Experimental Brain Research.
Cirio, G., Vangorp, P., Chapoulie, E., Marchal, M., L
´
ecuyer,
A., and Drettakis, G. (2012). Walking in a cube:
Novel metaphors for safely navigating large virtual
environments in restricted real workspaces. IEEE
Transactions on Visualization and Computer Graph-
ics, 18(4):546–554.
Crawford, J. R. and Howell, D. C. (1998). Comparing an in-
dividuals test score against norms derived from small
samples. The Clinical Neuropsychologist, 12(4):482–
486.
Cruz-Neira, C., Sandin, D., DeFanti, T., Kenyon, R., and
Hart, J. (1992). The cave: audio visual experience au-
tomatic virtual environment. Communications of the
ACM, 35(6):65–72.
Darken, R., Cockayne, W., and Carmein, D. (1997). The
omni-directional treadmill: A locomotion device for
virtual worlds. In Proceedings of of UIST 97.
DSouza, Pathirana, M. . A. (2011). Kinect to architec-
ture. In IVCNZ 201, pages 155–160, Auckland, New
Zealand.
Elbaz, J. N., Schenirderman, A., Klinger, E., and Shevil,
E. (2009). Using virtual reality to evaluate executive
functioning among persons with schizophrenia: A va-
lidity study. Schizophrenia Research, 115:270–277,
Fernandes, K. J., Raja, V., and Eyre, J. (2003). Cybersphere:
the fully immersive spherical projection system. Com-
munications of the ACM, 46(9):141–146.
Figueiredo, L. S., Pinheiro, M., Vilar Neto, E., Menezes, T.,
Teixeira, J. a. M., Teichrieb, V., Alessio, P., and Fre-
itas, D. (2014). In-place natural and effortless naviga-
tion for large industrial scenarios. In Proceedings of
the Third International Conference on Design, User
Experience, and Usability. User Experience Design
for Diverse Interaction Platforms and Environments
- Volume 8518, pages 550–561, New York, NY, USA.
Springer-Verlag New York, Inc.
Fischer, L., Oliveira, G., Osmari, D., and Nedel, L. (2011).
Finding hidden objects in large 3d environments: The
supermarket problem. In XIII Symposium on Virtual
Reality, pages 106–109.
Flynn, D., van Schaik, P., Blackman, T., Femcott, C.,
Hobbs, B., and Calderon, C. (2003). Developing a
virtual reality-based methodology for people with de-
mentia: a feasibility study. Cyberpsychology and Be-
haviour, 6:591–611,
Gaggioli, A., Keshner, E., Weiss, P., and Riva, G. (2009).
Advanced technologies in rehabilitation - empowering
cognitive, physical, social and communicative skills
through virtual reality, robots, wearable systems and
brain-computer interfaces. .
Hadad (2012). Rehabilitation tools along the reality contin-
uum: from mock-up to virtual interactive shopping to
a living lab. In 9th Intl Conf. Disability, Virtual Reality
& Associated Technologies (ICDVRAT), pages 47–52,
Laval, France.
Herrewijn, L., Poels, K., and Calleja, G. (2013). The
relationship between player involvement and immer-
sion: an experimental investigation. In 8
th
Inter-
national Conference on the Foundations of Digital
Games, page 4, Chania, Crete, Greece.
Iwata, H. (1999). The torus treadmill: Realizing locomotion
in ves. IEEE Computer Graphics and Applications,
19(6):30–35.
Iwata, H., Yano, H., Fukushima, H., and Noma, H. (2005).
Circulafloor: A locomotion interface using circulation
of movable tiles. In IEEE Virtual Reality Interna-
tional Conference (VR’2005), pages 223–230, Bohn,
Germany. IEEE Computer Society.
Josman, N., Hof, E., Klinger, E., Marie, R., Goldenberg, K.,
Weiss, P., and Kizony, R. (2006). Performance within
a virtual supermarket and its relationship to execu-
tive functions in post-stroke patients. In International
Workshop on Virtual Rehabilitation, pages 106–109.
Josman, N., Kizony, R., Hof, E., Goldenberg, K., Weiss,
P., and Klinger, E. (2014). Using the virtual action
planning-supermarket for evaluating executive func-
tions in people with stroke. J Stroke Cerebrovasc Dis,
23(5):879–887,
Josman, N., Klinger, E., and Kizony, R. (2008). Perfor-
mance within the virtual action planning supermarket
(vap-s): an executive function profile of three differ-
ent populations suffering from deficits in the central
nervous system. In ICDVRAT, pages 33–38, Maia,
Portugal.
Katz, N., Ring, H., Naveh, Y., Kizony, R., Feintuch, U., and
Weiss, P. (2005). Interactive virtual environment train-
ing for safe street crossing of right hemisphere stroke
patients with unilateral spatial neglect. Disability Re-
habilation, 27:1235–1243,
Klinger, E., Chemin, I., Lebreton, S., and Mari
´
e, R. (2003).
A virtual supermarket to assess cognitive planning.
Cyberpsychololgy and Behaviour, 7(3):292–293,
Klinger, E., Chemin, I., S, S. L., and Mari
´
e, R. M. (2006).
Virtual action planning in parkinson disease : Acon-
trol study. Cyberpsychology and Behaviour, 9:342–
347,
Lee, J., Ku, J., Cho, W., Hahn, W., Kim, I., and et al, S. L.
(2003). A virtual reality system for the assessment
and rehabilitation of the activities of daily living. Cy-
berpsychology and Behaviour, 6:383–388,
Mari
´
e, R. M., Klinger, E., Chemin, I., and Josset, M. (2003).
Cognitive planning assessed by virtual reality. In
Laval Virtual Conference (VRIC 2003), pages 119–
125, Laval, France.
McClymont, J., Shuralyov, D., and Stuerzlinger, W. (2011).
Comparison of 3d navigation interfaces. In VECIMS,
pages 7–12, l.
GRAPP 2016 - International Conference on Computer Graphics Theory and Applications
278