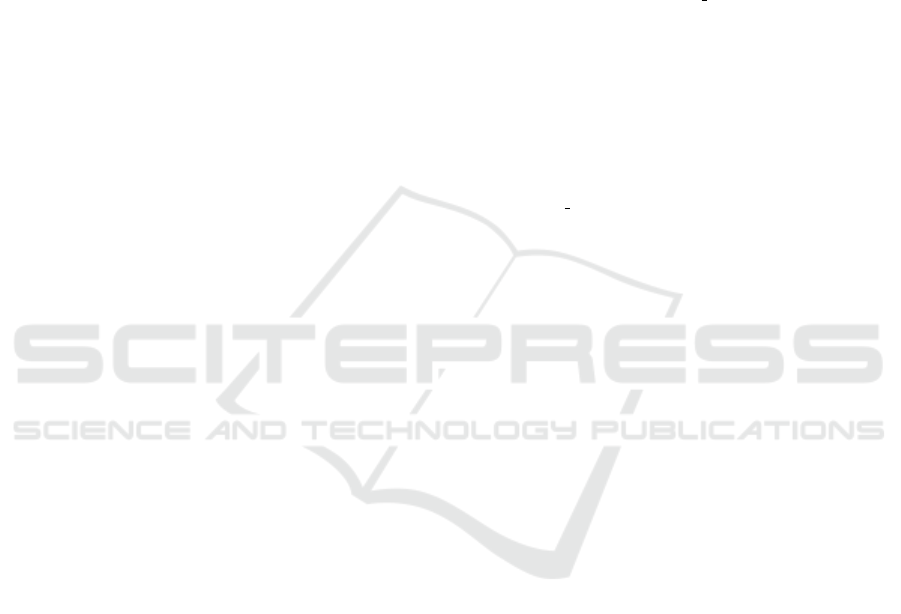
Grey, K. and Maguiness, S. (2016). Atopic dermatitis: Up-
date for pediatricians. Pediatric Annals, 45(8):e280–
286. PMID: 27517355.
Hardwicke, J. T., Osmani, O., and Skillman, J. M.
(2016). Detection of perforators using smartphone
thermal imaging. Plastic and Reconstructive Surgery,
137(1):39–41. PMID: 26710006.
Hilton-Jones, D. (2003). Diagnosis and treatment of inflam-
matory muscle diseases. Journal of Neurology, Neu-
rosurgery & Psychiatry, 74(suppl 2):ii25–ii31. PMID:
12754326.
Ju, X., Nebel, J., and Siebert, J. P. (2005). 3D thermogra-
phy imaging standardization technique for inflamma-
tion diagnosis. In Gong, H., Cai, Y., and Chatard, J.,
editors, Proceedings Volume 5640, Infrared Compo-
nents and Their Applications, page 266.
Krysko, D. V., Vanden Berghe, T., D’Herde, K., and Van-
denabeele, P. (2008). Apoptosis and necrosis: detec-
tion, discrimination and phagocytosis. Methods (San
Diego, Calif.), 44(3):205–221. PMID: 18314051.
Kunii, T. L. (2012). Frontiers in Computer Graphics: Pro-
ceedings of Computer Graphics Tokyo 84. Springer
Science & Business Media. Google-Books-ID: YYyr-
CAAAQBAJ.
Lacarrubba, F., Pellacani, G., Gurgone, S., Verz, A. E., and
Micali, G. (2015). Advances in non-invasive tech-
niques as aids to the diagnosis and monitoring of ther-
apeutic response in plaque psoriasis: a review. Inter-
national Journal of Dermatology, 54(6):626–634.
Lazarous, M. C. and Kerdel, F. A. (2002). Topical
tacrolimus protopic. Drugs of Today (Barcelona,
Spain: 1998), 38(1):7–15. PMID: 12532181.
Lipsky, B. A., Berendt, A. R., Deery, H. G., Embil, J. M.,
Joseph, W. S., Karchmer, A. W., LeFrock, J. L., Lew,
D. P., Mader, J. T., Norden, C., and Tan, J. S. (2004).
Diagnosis and treatment of diabetic foot infections.
Clinical Infectious Diseases, 39(7):885–910. PMID:
15472838.
Lu, G. and Fei, B. (2014). Medical hyperspectral imaging: a
review. Journal of Biomedical Optics, 19(1):010901–
010901.
Micro-Epsilon (2008). Instruction manual scanCONTROL.
http://www.micro-epsilon.cz/download/manuals/man–
scanCONTROL-2700–en.pdf.
Necas, M. (2011). Atopicky ekzem. Ceska dermatoven-
erologie, 1(2):8–20.
Nedorost, S. (2012). Generalized dermatitis in clinical
practice. Springer, Dordrecht.
Nejdl, L., Kudr, J., Ruttkay-Nedecky, B., Heger, Z., Zima,
L., Zalud, L., Krizkova, S., Adam, V., Vaculovicova,
M., and Kizek, R. (2015). Remote-Controlled robotic
platform for electrochemical determination of water
contaminated by heavy metal ions. International Jour-
nal of Electrochemical Science, 10(4):3635–3643.
WOS:000352222500069.
Palencar, R., Vdolecek, F., and Halaj, M. (2001). Nejis-
toty v mereni ii: nejistoty primych mereni. Automa,
2001(10):55–56.
Ring, E. F. J. and Ammer, K. (2012). Infrared thermal
imaging in medicine. Physiological Measurement,
33(3):R33.
Smith, K. B. and Zheng, Y. F. (1998). Accuracy analysis
of point laser triangulation probes using simulation.
Journal of Manufacturing Science and Engineering,
120(4):736–745.
Sprikkelman, A. B., Tupker, R. A., Burgerhof, H.,
Schouten, J. P., Brand, P. L., Heymans, H. S., and van
Aalderen, W. M. (1997). Severity scoring of atopic
dermatitis: a comparison of three scoring systems. Al-
lergy, 52(9):944–949. PMID: 9298180.
Suffern, K. (2016). Ray Tracing from the Ground Up. CRC
Press. Google-Books-ID: RDYCwAAQBAJ.
Tollefson, M. M., Bruckner, A. L., and Section On Der-
matology (2014). Atopic dermatitis: skin-directed
management. Pediatrics, 134(6):e1735–1744. PMID:
25422009.
Vardasca, R. and Simoes, R. (2013). Current issues in med-
ical thermography. In Topics in Medical Image Pro-
cessing and Computational Vision, Lecture Notes in
Computational Vision and Biomechanics, pages 223–
237. Springer, Dordrecht. DOI: 10.1007/978-94-007-
0726-9 12.
Varothai, S., Nitayavardhana, S., and Kulthanan, K. (2013).
Moisturizers for patients with atopic dermatitis. Asian
Pacific Journal of Allergy and Immunology, 31(2):91–
98. PMID: 23859407.
Xenics (2009). Xenics GOBI 1954 reference manual.
Zalud, L. (2006). ARGOS - system for heterogeneous mo-
bile robot teleoperation. In 2006 IEEE/RSJ Interna-
tional Conference on Intelligent Robots and Systems,
IROS 2006, pages 211–216, Beijing; China.
Zalud, L., Kocmanova, P., Burian, F., Jilek, T., Kalvoda, P.,
and Kopecny, L. (2015). Calibration and evaluation of
parameters in a 3D proximity rotating scanner. Elek-
tronika ir Elektrotechnika, 21(1):3–12.
Multispectral 3D Surface Scanning System RoScan and its Application in Inflammation Monitoring and Quantification
113