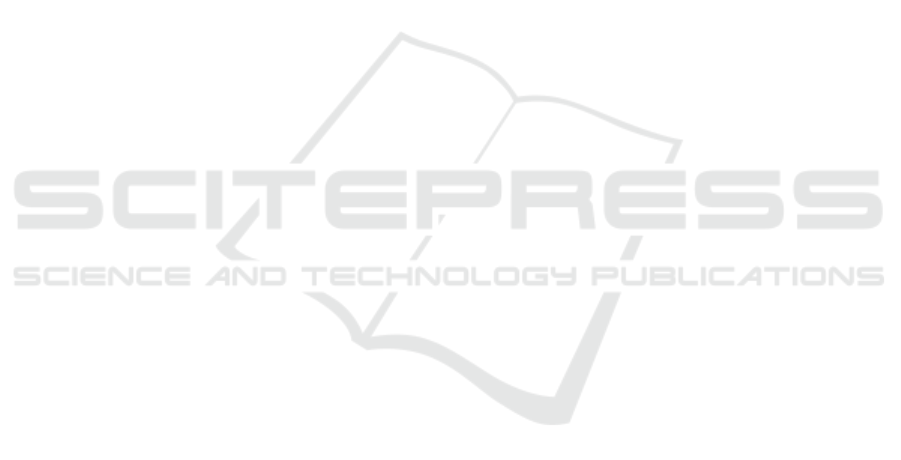
The 3D-printing of the mechanical prototype de-
scribed in section 2.5 is expected to be further devel-
oped in future work, so that the implementation of the
optical system can be performed. After the prototype
construction, several ”in vivo” tests are expected.
A light hazard measurement, regarding the ISO
15004-2 (ISO 15004-2:2007, 2007) and ISO 10940
(ISO 10940:2009, 2009) norms, for this kind of in-
struments is also mandatory, being the first for light
hazard protection of Ophthalmologic Instruments and
the second specifically for Fundus Cameras.
ACKNOWLEDGEMENTS
We would like to acknowledge the financial support
obtained from North Portugal Regional Operational
Programme (NORTE 2020), Portugal 2020 and the
European Regional Development Fund (ERDF) from
European Union through the project Symbiotic tech-
nology for societal efficiency gains: Deus ex Machina
(DEM), NORTE-01-0145-FEDER-000026.
REFERENCES
Atchison, D. and Smith, G. (2000). Optics of the Human
Eye. Butterworth-Heinemann, page 259.
Benbassat, J., Polak, B. C. P., and Javitt, J. C. (2012). Ob-
jectives of teaching direct ophthalmoscopy to medical
students. Acta Ophthalmologica, 90(6):503–507.
Born, M. and Wolf, E. (1999). Principles of Optics (7th
Ed). Cambridge University Press.
Bunce, C. and Wormald, R. (2006). Leading causes of cer-
tification for blindness and partial sight in England &
Wales. BMC public health, 6:58.
Cheung, N., Mitchell, P., and Wong, T. Y. (2010). Diabetic
retinopathy. The Lancet, 376(9735):124–136.
Cunha-Vaz, J. (2007). Characterization and relevance of
different diabetic retinopathy phenotypes. Develop-
ments in Ophthalmology, 39:13–30.
D-EYE S.r.l. D-EYE Ophthalmoscope.
do Prado, R. S., Figueiredo, E. L., and Magalhaes, T. V. B.
(2002). Retinal detachment in preeclampsia. Arquivos
brasileiros de cardiologia, 79(2):183–186.
Giancardo, L. (2012). Automated fundus images analysis
techniques to screen retinal diseases in diabetic pa-
tients Docteur de l ’ universit
´
e Automated Fundus Im-
ages Analysis Techniques to Screen Retinal Diseases
in Diabetic Patients.
ISO 10940:2009 (2009). Ophthalmic instruments - Fun-
dus cameras. Standard, International Organization for
Standardization, Geneva, CH.
ISO 15004-2:2007 (2007). Ophthalmic instruments - Fun-
damental requirements and test methods.Part 2: Light
Hazard Protection. Standard, International Organiza-
tion for Standardization, Geneva, CH.
Jenkins, F. and White, H. (1957). Fundamentals of optics.
McGraw-Hill.
Jin, K., Lu, H., Su, Z., Cheng, C., Ye, J., and Qian, D.
(2017). Telemedicine screening of retinal diseases
with a handheld portable non-mydriatic fundus cam-
era. BMC Ophthalmology, 17(1):89.
Kauppi, T. (2010). Eye Fundus Image Analysis for Auto-
matic Detection of Diabetic Retinopathy.
Li, H., Esquivel, A., Davis, G., and Krupinski, E. (2006).
Evaluation of digital resolution for viewing diabetic
retinopathy microaneurysms.
Patton, N., Aslam, T., MacGillivray, T., Pattie, A., Deary,
I. J., and Dhillon, B. (2005). Retinal vascular image
analysis as a potential screening tool for cerebrovas-
cular disease: a rationale based on homology be-
tween cerebral and retinal microvasculatures. J Anat,
206(4):319–348. 15817102[pmid].
P
´
erez, M. A., Bruce, B. B., Newman, N. J., and Biousse,
V. (2012). The use of retinal photography in non-
ophthalmic settings and its potential for neurology.
Neurologist, 18(6):350–355. 23114666[pmid].
Phillips, C. I. (1984). Dilate the pupil and see the fun-
dus. Br Med J (Clin Res Ed), 288(6433):1779–1780.
6428541[pmid].
Quellec, G., Bazin, L., Cazuguel, G., Delafoy, I., Coch-
ener, B., and Lamard, M. (2016). Suitability of a low-
cost, handheld, nonmydriatic retinograph for diabetic
retinopathy diagnosis. Translational Vision Science &
Technology, 5(2):16.
Roorda, A. and Duncan, J. L. (2015). Adaptive op-
tics ophthalmoscopy. Annu Rev Vis Sci, 1:19–50.
26973867[pmid].
Salz, D. A. and Witkin, A. J. (2015). Imaging in diabetic
retinopathy. Middle East African journal of ophthal-
mology, 22(2):145.
Shen, B. Y. and Mukai, S. (2017). A portable, inexpensive,
nonmydriatic fundus camera based on the raspberry
pi
R
computer. Journal of Ophthalmology, 2017(3):5.
Swedish, T., Roesch, K., Lee, I., Rastogi, K., Bernstein, S.,
and Raskar, R. (2015). eyeselfie: Self directed eye
alignment using reciprocal eye box imaging. ACM
Trans. Graph., 34(4).
Tarr, J. M., Kaul, K., Chopra, M., Kohner, E. M., and Chib-
ber, R. (2013). Pathophysiology of Diabetic Retinopa-
thy. ISRN Ophthalmology, 2013:1–13.
Tocci, M. (2007). How to model the human eye in zemax.
Tran, K., Mendel, T. A., Holbrook, K. L., and Yates, P. A.
(2012). Construction of an inexpensive, hand-held
fundus camera through modification of a consumer
”point-and-shoot” camera. Investigative ophthalmol-
ogy & visual science, 53 12:7600–7.
Volk Optical Inc. Volk InView.
Volk Optical Inc. Volk Pictor Plus.
BIODEVICES 2018 - 11th International Conference on Biomedical Electronics and Devices
70