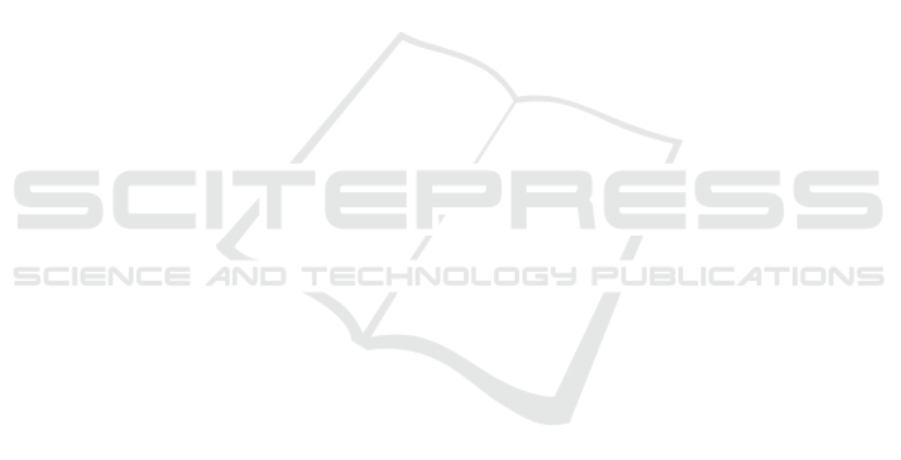
ACKNOWLEDGEMENTS
This work was performed within the Project No.
2013/11/B/ST8/03672 sponsored by the National
Science Centre (NCN) of Poland.
REFERENCES
Abe T., Sakane M., Ikoma T., Kobayashi M., Nakamura S.,
Ochiai N., 2008. Intraosseous delivery of paclitaxel-
loaded hydroxyapatitealginate composite beads
delaying paralysis caused by metastatic spine cancer
in rats. J. Neurosurg. Spine 9 , 502–510.
Agrawal V., Doelken P., Sahn S.A., 2008. Pleural Fluid
Analysis in Chylous Pleural Effusion. CHEST Journal.
133(6), 1436-1441.
Al-Ghananeem A.M., Malkawi A.H., Muammer Y.M.,
Balko J.M., Black E.P., Mourad W., Romond E.,
2009. Intratumoral delivery of Paclitaxel in solid tumor
from biodegradable hyaluronan nanoparticle
formulations. AAPS PharmSciTech. 10, 410–417.
Barhoumi A., Salvador‐Culla B., Kohane D.S., 2015. NIR‐
Triggered Drug Delivery by Collagen‐Mediated
Second Harmonic Generation. Advanced healthcare
materials. 4(8), 1159-1163.
Davis M.E., Chen Z., Shin D.M., 2008. Nanoparticle
therapeutics: an emerging treatment modality for
cancer. Nat. Rev. Drug Discov. 7, 771–782.
De Souza R., Zahedi P., Allen C.J., Piquette-Miller M.,
2010. Polymeric drug delivery systems for localized
cancer chemotherapy. Drug Deliv. 17, 365–375.
Gerber D.E., Gallia G.L., Tyler B.M., Eberhart C.G., Royer
G., Grossman S.A., 2011. A novel polymer gel for the
delivery of local therapies to intracranial tumors: In
vivo safety evaluation. J. Biomed. Mater. Res. A. 99,
479–484.
Jacobs N.J., Van Denmark P., 1960. The purification and
properties of the α-glycerophosphate-oxidizing enzyme
of Streptococcus faecalis 10C1. J. Arch. Biochem.
Biophys. 88, 250-255.
Karpińska-Jazdon L., Spychalski W., 2005. Diagnostic
difficulties in case of pleural and chylous effusion in
mesothelioma patient. Wielkopolskie Centrum Chorób
Płuc I Gruźlicy w Poznaniu, Współczesna Onkologia
tom 9, 273-275.
Klinker K., 2017, Secondary-Structure-Driven Self-
Assembly of Reactive Polypept(o)ides: Controlling
Size, Shape, and Function of Core Cross-Linked
Nanostructures, Angewandte Chemie International
Edition, 56, 9608-9613.
Konishi M., Tabata Y., Kariya M., Suzuki A., Mandai M.,
Nanbu K., Takakura K., Fujii S., 2003. In vivo anti-
tumor effect through the controlled release of cisplatin
from biodegradable gelatin hydrogel. J. Control.
Release. 92, 301–313.
Kulkarni C. V., Moinuddin Z., Patil-Sen Y., Littlefield R.,
Hood M., 2015. Lipid-hydrogel films for sustained drug
release. International journal of pharmaceutics. 479(2),
416-421.
Langer R., 1983. Implantable controlled release systems.
Pharmacol. Ther. 21, 35–51.
Li X., Kong X., Zhang J., Wang Y., Wang Y., Shi S., Guo
G., Luo F., Zhao X., Wei Y., Qian Z., 2011. A novel
composite hydrogel based on chitosan and inorganic
phosphate for local drug delivery of camptothecin
nanocolloids. J. Pharm. Sci. 100, 232–241.
Li X., Lachmanski L., Safi S., Sene S., Serre C., Greneche
J., Zhang J., Gref R., 2017, New insights into the
degradation mechanism of metal-organic frameworks
drug carriers, Scientific Reports 7, 13142- 13152.
Liu X., Heng W.S., Li Q., Chan L.W. 2006. Novel
polymeric microspheres containing norcantharidin for
chemoembolization. J. Control. Release. 116, 35–41.
Lowry O., Rosebrough N., Farr A., Randall R., 1951.
Protein measurement with the Folin phenol reagent. J.
Biol. Chem. 193, 265– 270.
McDaniel J.R., Callahan D.J., Chilkoti A., 2010. Drug
delivery to solid tumors by elastin-like polypeptides.
Adv. Drug Deliv. Rev. 62, 1456–1467.
Miller C.N., 1959. Use of dinitrosalicyle acid reagent for
determination of reducing sugar. Analit. Chem. 81, 426
– 428.
Trusek-Holownia A., Noworyta A., 2003, Modelling of the
enzymatic synthesis of taste dipeptides with
simultaneous extraction in a membrane phase
contactor, Desalination 162, 335-342.
Trusek-Holownia A., Jaworska P., 2014. Polymeric drug
carriers, Biocybern. Biomed. Eng. 35, 192–197.
Weinberg B.D., Blanco E., Gao J., 2008. Polymer implants
for intratumoral drug delivery and cancer therapy. J.
Pharm. Sci. 97, 1681–1702.
Wolinsky J. B., Colson Y. L., Grinstaff M. W., 2012. Local
drug delivery strategies for cancer treatment: gels,
nanoparticles, polymeric films, rods, and wafers.
Journal of controlled release. 159(1), 14-26.
BIODEVICES 2018 - 11th International Conference on Biomedical Electronics and Devices
246