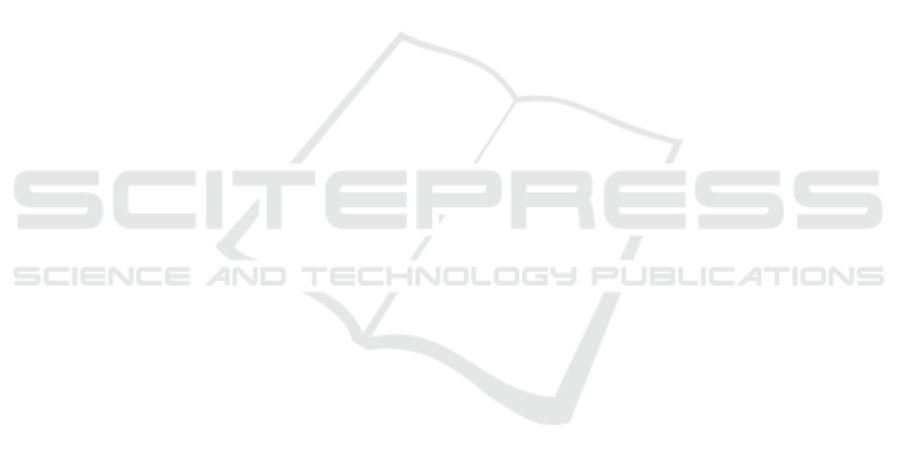
Document]. URL https://www.automotiveworld.com
/news-releases/new-audi-e-gas-offer-standard-80-
percent-lower-co2-emissions/ (accessed 10.21.17).
Batel, S., Devine-Wright, P., Tangeland, T., 2013. Social
acceptance of low carbon energy and associated
infrastructures: A critical discussion. Energy Policy 58,
1–5. doi:10.1016/j.enpol.2013.03.018.
Bayer Material Science, n.d. Bayer’s CO2 projects Use of
carbon dioxide for the production of plastics [WWW
Document].
Castellani, V., Sala, S., Benini, L., 2017. Hotspots analysis
and critical interpretation of food life cycle assessment
studies for selecting eco-innovation options and for
policy support. J. Clean. Prod., Towards eco-efficient
agriculture and food systems: selected papers
addressing the global challenges for food systems,
including those presented at the Conference “LCA for
Feeding the planet and energy for life” (6-8 October
2015, Stresa & Milan Expo, Italy) 140, Part 2, 556–568.
doi:10.1016/j.jclepro.2016.05.078.
Coates, G.W., Moore, D.R., 2004. Diskrete
Metallkatalysatoren zur Copolymerisation von CO2 mit
Epoxiden: Entdeckung, Reaktivität, Optimierung,
Mechanismus. Angew. Chem. 116, 6784–6806.
doi:10.1002/ange.200460442.
Covestro, 2016. Covestro starts brand launch for CO2
products [WWW Document]. URL
http://press.covestro.com/news.nsf/id/Covestro-starts-
brand-launch-for-CO2-products.
International Fertilizer Industry Association, 2009.
Fertilizers, Climate Change and Enhancing
Agricultural Productivity Sustainably [WWW
Document]. URL http://www.fertilizer.org/imis20/
images/Library_Downloads/2009_ifa_climate_change.
pdf?WebsiteKey=411e9724-4bda-422f-abfc-
8152ed74f306&=404%3bhttp%3a%2f%2fwww.fertili
zer.org%3a80%2fen%2fimages%2fLibrary_Download
s%2f2009_ifa_climate_change.pdf (accessed 8.26.15).
Jones, C., Olfe-Kräutlein, B., Kaklamanou, D., 2016. Lay
perceptions of Carbon Dioxide Utilization (CDU)
technologies in the UK and Germany: A qualitative
interview study. Presented at the 4th International
Conference on Carbon Dioxide Utilisation (ICCDU),
Sheffield, United Kingdom.
Jones, C.R., 2015. Chapter 15 - Understanding and
Assessing Public Perceptions of Carbon Dioxide
Utilisation (CDU) Technologies, in: Styring, P.,
Quadrelli, E.A., Armstrong, K. (Eds.), Carbon Dioxide
Utilisation: Closing the Carbon Cycle. Elsevier,
Amsterdam, pp. 273–283.
Jones, C.R., Olfe-Kraeutlein, B., Naims, H., Armstrong, K.,
2017. The social acceptance of carbon dioxide
utilisation: A review and research agenda. Front.
Energy Res. 5, 11.
Kätelhön, A., Bardow, A., Suh, S., 2016. Stochastic
Technology Choice Model for Consequential Life
Cycle Assessment. Environ. Sci. Technol. 50, 12575–
12583.
Markewitz, P., Kuckshinrichs, W., Leitner, W., Linssen, J.,
Zapp, P., Bongartz, R., Schreiber, A., Müller, T.E.,
2012. Worldwide innovations in the development of
carbon capture technologies and the utilization of CO 2.
Energy Environ. Sci. 5, 7281–7305.
Perdan, S., Jones, C.R., Azapagic, A., 2017. Public
awareness and acceptance of carbon capture and
utilisation in the UK. Sustain. Prod. Consum. 10, 74–
84. doi:10.1016/j.spc.2017.01.001.
Rao, V.R., 2014. Applied Conjoint Analysis. Springer
Berlin Heidelberg, Berlin, Heidelberg.
Rebitzer, G., Ekvall, T., Frischknecht, R., Hunkeler, D.,
Norris, G., Rydberg, T., Schmidt, W.-P., Suh, S.,
Weidema, B.P., Pennington, D.W., 2004. Life cycle
assessment: Part 1: Framework, goal and scope
definition, inventory analysis, and applications.
Environ. Int. 30, 701–720.
Sawtooth Software, 2013. The CBC System for Choice-
Based Conjoint Analysis (2013) Technical Paper
Version 8 [WWW Document]. URL https://
sawtoothsoftware.com/download/techpap/cbctech.pdf
(accessed 8.20.15).
Styring, P., Quadrelli, E.A., Armstrong, K. (Eds.), 2015.
Carbon Dioxide Utilisation: Closing the Carbon Cycle.
Elsevier.
van Heek, J., Arning, K., Ziefle, M., 2017a. Differences
between Laypersons and Experts in Perceptions and
Acceptance of CO2-utilization for Plastics Production.
Energy Procedia, 13th International Conference on
Greenhouse Gas Control Technologies, GHGT-13, 14-
18 November 2016, Lausanne, Switzerland 114, 7212–
7223. doi:10.1016/j.egypro.2017.03.1829.
van Heek, J., Arning, K., Ziefle, M., 2017b. Reduce, reuse,
recycle: Acceptance of CO2-utilization for plastic
products. Energy Policy 105, 53–66.
doi:10.1016/j.enpol.2017.02.016.
von der Assen, N., Jung, J., Bardow, A., 2013. Life-cycle
assessment of carbon dioxide capture and utilization:
avoiding the pitfalls. Energy Environ. Sci. 6, 2721–
2734.von der Assen, N., Bardow, A., 2014. Life cycle
assessment of polyols for polyurethane production
using CO2 as feedstock: insights from an industrial case
study. Green Chem. 16, 3272–3280.
doi:10.1039/C4GC00513A.
von der Assen, N., Voll, P., Peters, M., Bardow, A., 2014.
Life cycle assessment of CO2 capture and utilization: a
tutorial review. Chem. Soc. Rev. 43, 7982–7994.
doi:10.1039/C3CS60373CWallquist, L., Visschers,
V.H.M., Dohle, S., Siegrist, M., 2012. The Role of
Convictions and Trust for Public Protest Potential in the
Case of Carbon Dioxide Capture and Storage (CCS).
Hum. Ecol. Risk Assess. Int. J. 18, 919–932.
doi:10.1080/10807039.2012.688719.
Wilson, G., Travaly, Y., Brun, T., Knippels, H., Armstrong,
K., Styring, P., Krämer, D., Saussez, G., Bolscher, H.,
2015. A Vision for Smart CO 2 Transformation in
Europe: Using CO 2 as a Resource. SCOT Project.
Wüstenhagen, R., Wolsink, M., Bürer, M.J., 2007. Social
acceptance of renewable energy innovation: An
introduction to the concept. Energy Policy 35, 2683–
2691.
SMARTGREENS 2018 - 7th International Conference on Smart Cities and Green ICT Systems
42