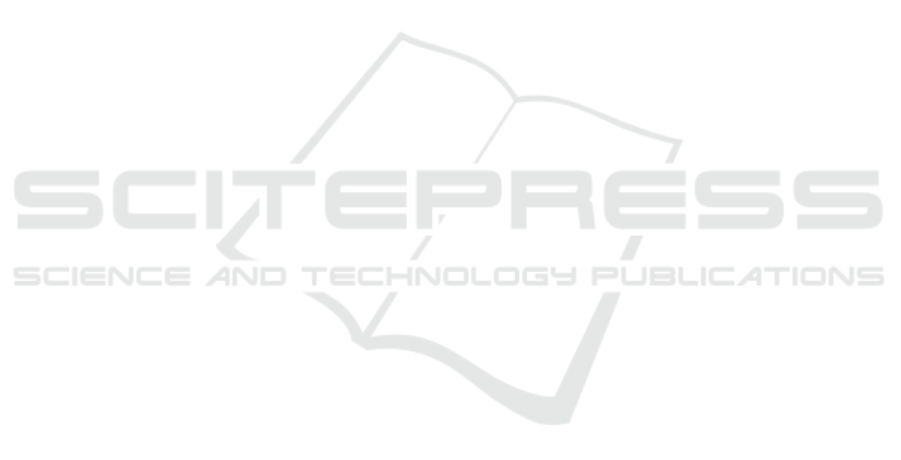
visual size. In Perception & Psychophysics, 68(7),
1191-1203.
Gardner, E. P., Spencer, W. A., 1972. Sensory Funneling.
I. Psychophysical Observations of Human Subjects
and Responses of Cutaneous in Mechanoreceptive
Afferents in the Cat to Patterned Skin Stimuli. In
Journal of Neurophysiology, 35(6), 925–953.
Geldard, F. A., Sherrick, C. E., 1972. The Cutaneous
“Rabbit”: A Perceptual Illusion. In Science,
178(4057), 178–179.
Gentaz, E., Hatwell, Y., 2004. Geometrical haptic
illusions: The role of exploration in the Müller-Lyer,
vertical-horizontal, and Delboeuf illusions. In
Psychonomic Bulletin and Review, 11(1), 31–40.
Hayward, V., 2015. Tactile illusions. In Scholarpedia,
10(3), 8245.
Hoffmann, R., Spagnol, S., Kristjánsson, Á., Unnthorsson,
R., 2018. Evaluation of an Audio-Haptic Sensory
Substitution Device for Enhancing Spatial Awareness
for the Visually Impaired. In Optometry and Vision
Science, 95(9), 757-765.
Jack, R., McPherson, A., Stockman, T., 2015. Designing
Tactile Musical Devices with and for Deaf Users: a
Case Study. In Proceedings of the International
Conference on the Multimedia Experience of Music, 3-
25.
Jamal, Y., Lacey, S., Nygaard, L., Sathian, K., 2017.
Interactions between Auditory Elevation, Auditory
Pitch and Visual Elevation during Multisensory
Perception. In Multisensory Research, 30(3–5), 287–
306.
Jóhannesson, Ó. I., Hoffmann, R., Valgeirsdóttir, V. V.,
Unnþórsson, R., et al., 2017. Relative vibrotactile
spatial acuity of the torso. In Experimental Brain
Research, 235(11), 3505–3515.
Karam, M., Nespoli, G., Russo, F., Fels, D. I., 2009.
Modelling perceptual elements of music in a
vibrotactile display for deaf users: A field study. In
Second International Conferences on Advances in
Computer-Human Interactions. IEEE.
Kristjánsson, Á., Moldoveanu, A., Jóhannesson, Ó. I.,
Balan, O. et al., 2016. Designing sensory-substitution
devices: Principles, pitfalls and potential. In
Restorative Neurology and Neuroscience, 34(5), 769–
787.
Lechelt, E. C., Borchert, R., 1977. The interdependence of
time and space in somesthesis: The Tau effect
reexamined. In Bulletin of the Psychonomic Society,
10(3), 191–193.
Lederman, S. J., Jones, L. A., 2011. Tactile and Haptic
Illusions. In IEEE Transactions on Haptics, 4(4), 273–
294.
Mahzoun, E. E., 2013. Good Vibrations : A vibrotactile
aid toward music sensation aiming at helping deaf
people. Blekinge Institute of Technology.
Martino, G. Marks, L. E., 2000. Cross-modal interaction
between vision and touch: the role of synesthetic
correspondence. In Perception, 29, 745–754.
Melara, R. D., 1989. Dimensional interaction between
color and pitch. In Journal of Experimental
Psychology: Human Perception and Performance,
15(1), 69.
Nanayakkara, S. C., Wyse, L., Ong, S. H., Taylor, E. A.,
2013. Enhancing musical experience for the hearing-
impaired using visual and haptic displays. Human-
Computer Interaction, 28(2), 115–160.
Nava, E., Grassi, M., Turati, C., 2016. Audio-visual,
visuo-tactile and audio-tactile correspondences in pre-
schoolers. In Multisensory Research, 29(1–3), 93–111.
Novich, S. D., Eagleman, D. M., 2015. Using space and
time to encode vibrotactile information: toward an
estimate of the skin’s achievable throughput. In
Experimental Brain Research, 233(10), 2777–2788.
Occelli, V., Spence, C., Zampini, M., 2009. Compatibility
effects between sound frequency and tactile elevation.
In NeuroReport, 20(8), 793–797.
Olejnik, S., Algina, J., 2003. Generalized Eta and Omega
Squared Statistics: Measures of Effect Size for Some
Common Research Designs. In Psychological
Methods, 8(4), 434–447.
Peirce, J. W., 2009. Generating stimuli for neuroscience
using PsychoPy. In Frontiers in neuroinformatics, 2-
10.
Peters, A., 2002. The effects of normal aging on myelin
and nerve fibers: a review. In Journal of
neurocytology, 31(8-9), 581–593.
Precision Micordrives, 2018. ERM Vibration Motor.
https://www.precisionmicrodrives.com/product/307-
103-9mm-vibration-motor-25mm-type.
Accessed 02.05.2018.
Reich, L., Maidenbaum, S., Amedi, A., 2012. The brain as
a flexible task machine: Implications for visual
rehabilitation using noninvasive vs. invasive
approaches. In Current Opinion in Neurology, 25(1),
86–95.
Jones, L. A., Sofia, K., 2012. Measuring surface wave
propagation during vibrotactile stimulation. In Haptics
Symposium. IEEE.
Sorgini, F., Caliò, R., Carrozza, M. C., Oddo, C. M., 2018.
Haptic-assistive technologies for audition and vision
sensory disabilities. In Disability and Rehabilitation:
Assistive Technology, 13(4), 394-421.
Spence, C., 2011. Crossmodal correspondences: A tutorial
review. In Attention, Perception, and Psychophysics,
73(4), 971–995.
Stevens, J. C., Patterson, M. Q., 1995. Dimensions of
spatial acuity in the touch sense: Changes over the life
span. Somatosensory & motor research, 12(1), 29-47.
Striem-Amit, E., Bubic, A., Amedi, A., 2012.
Neurophysiological Mechanisms Underlying Plastic
Changes and Rehabilitation following Sensory Loss in
Blindness and Deafness. In Murray, M., MT W.
(Eds.), The Neural Bases of Multisensory Processes,
395–422. CRC Press/Taylor and Francis.
Van Erp, J. B. F., 2005. Vibrotactile spatial acuity on the
torso: Effects of location and timing parameters. 1st
Joint Eurohaptics Conference and Symposium on
Haptic Interfaces for Virtual Environment and
Teleoperator Systems. IEEE.
CHIRA 2018 - 2nd International Conference on Computer-Human Interaction Research and Applications
52