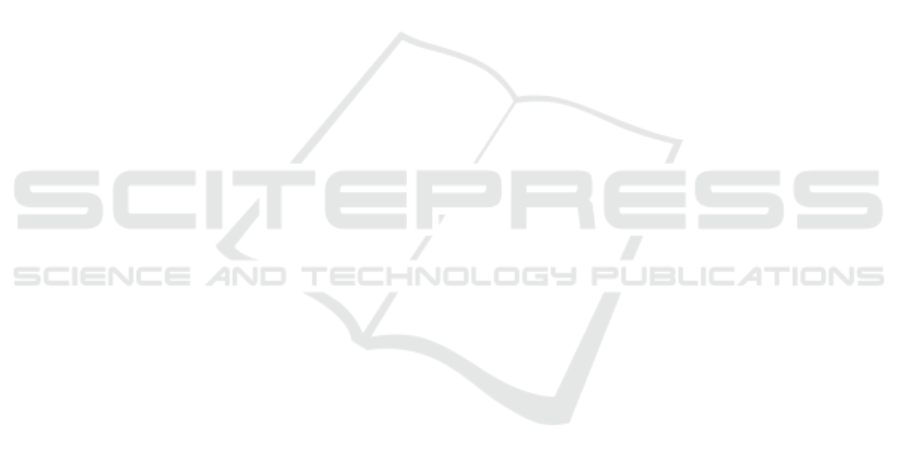
Energy Analysis and Exergy Analysis of a New Type Solar Air
Conditioning System
School of Aeronautic Science and Engineering, Beihang University, XueYuan Road, Beijing, China
Baixi_buaa@163.com
Keywords: Solar air conditioning, Heat pump, Energy Analysis, Exergy Analysis.
Abstract: This paper presents a novel type of solar air conditioning system. The steady thermodynamic analysis model
and exergy analysis model are given to analyse the performance of the novel system. The energy analysis and
exergy analysis are carried out through detailed calculation. The exergy system analysis shows that the highest
exergy destruction occurs in condensation-generation and condensation 2, which can be reduced with
parameter optimization. A range of operating conditions are selected and simulated for the novel solar air
conditioning system by changing the corresponding parameters while keeping other parameters consistent
with the design conditions. Compared with the original solar air conditioning system, the novel solar air
conditioning system possesses the advantages of low requirements for the performance of solar collectors and
smaller area of solar collectors. The novel system is also less affected by weather conditions.
1 INTRODUCTION
To reduce environment pollution and energy
conservation, solar air conditioning researches are of
great significance. Solar energy is a kind of clean and
renewable energy. Since the refrigerating demand and
the supply of solar radiation are almost in phase with
each other, the solar air conditioning system is
appealing to many researchers (Hwang, Y.,
Radermacher, R., Alili, A. and Kubo, I. 2008; Sekret,
R. and Turski, M. 2012 ). The most common kind of
solar air conditioning system, which can be seen as
the original system, is composed of solar collectors
and the single effect LiBr–H
2
O absorption chiller.
However, the original system is confronted with
many problems in practical operation, including
crucial problems like intermittency and instability. In
order to solve the existing problems, a number of
relevant studies are carried out by many researchers.
Xu presented a new solar powered absorption
refrigeration system with advanced energy storage
technology. The energy collected from the solar
radiation was transformed into the chemical potential
of the working fluid and stored in order to solve the
problem of the unconformity between solar radiation
and cooling demand (Xu, S., Huang, X. and Du, R.
2011). Lass-Seyoum tested a similar closed thermo-
chemical heat storage system. This method offers
several advantages including the possibility of long-
term storage with minimal thermal losses and a high-
energy storage density compared with sensible and
latent thermal storage principles (Lass-Seyoum, A.,
Blicker, M., Borozdenko, D., Friedrich, T. and
Langhof, T. 2012).
Ahachad combined an absorption heat pump
system and an absorption refrigeration system to form
a two-stage vapor absorption system. And the tests
conducted in Rabat (Morocco) showed that the
system can be operated at lower heat source
temperatures by using flat-plate collectors (Ahachad,
M., Charia, M. and Bernatchou, A. 1993). Jain
proposed a cascaded vapour compression–absorption
system (CVCAS) which consists of a vapour
compression refrigeration system (VCRS) coupled
with single effect vapour absorption refrigeration
system (VARS). Based on first and second laws, a
comparative performance analysis of CVCAS and an
independent VCRS has been carried out (Jain, V.,
Kachhwaha, S. and Sachdeva, G. 2013).
Prasartkaew studied the performance of a
renewable energy (solar–biomass) based on single
effect LiBr–H
2
O absorption chiller. The chiller and
overall system coefficient of performances were
found to be 0.7 and 0.55 respectively and the biomass
(charcoal) consumption for 24h operation was
24.44kg/day (Prasartkaew, B. and Kumar, S. 2010).
Y.L. Liu presented the performance prediction of a
solar/gas driving double effect LiBr-H
2
O absorption
Bai, X.
Energy Analysis and Exergy Analysis of a New Type Solar Air Conditioning System.
In 3rd International Conference on Electromechanical Control Technology and Transportation (ICECTT 2018), pages 33-40
ISBN: 978-989-758-312-4
Copyright © 2018 by SCITEPRESS – Science and Technology Publications, Lda. All rights reserved
33