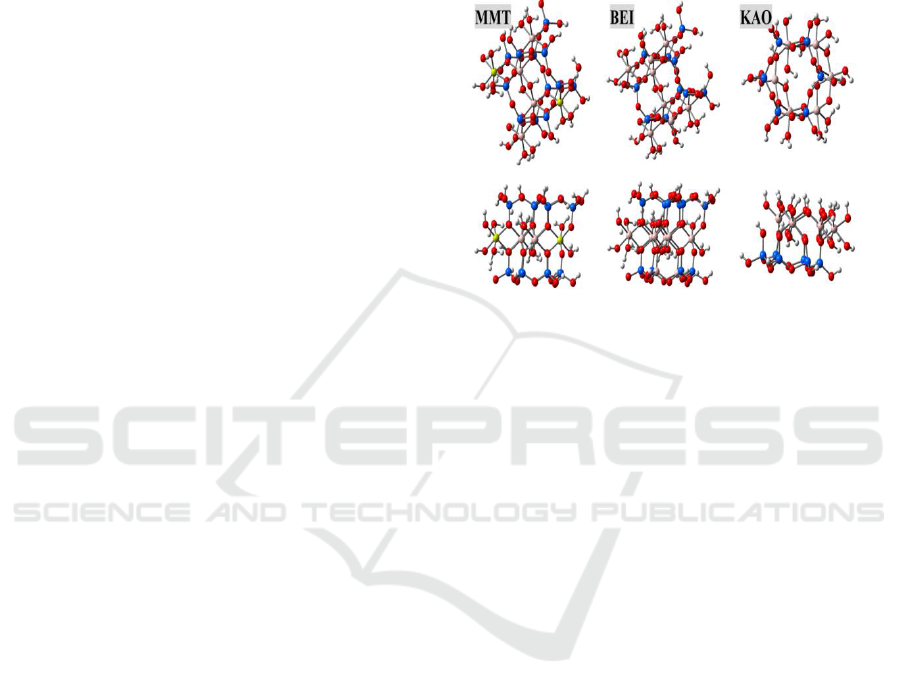
calculations were presently employed to study the
adsorption of K
+
ions at montmorillonite surfaces. In
addition to the adsorption of a single K
+
ion at clay
minerals with different negative charges as reported
previously (Wang et al., 2017; Li et al., 2017),
disparate numbers of K
+
ions (n = 13) were
considered to inspect the correlation between the
adsorption performances vs. the number of metal
ions. As observed experimentally, more than one
metal ion should be bound onto clay surfaces at the
same time. A portion of these adsorbed metal ions
can be exchanged and the exchange degree should
depend on the identities of two metal ions involved
in the exchange processes. The second issue of this
work was to address the exchange thermodynamics
among the various alkali ions on three clay surfaces
(montmorillonite, kaolinite, and beidellite). Then the
mechanism of cation exchange at clay surfaces was
tentatively proposed. Finally, the cation exchange
equilibrium constants for the various alkali ions at
clay surfaces were calculated and compare with the
experimental results.
2 COMPUTATIONAL SECTION
First-principles density functional theory
calculations were performed using Gaussian09
software packages (Frisch et al., 2013). Figure 1
showed the models for montmorillonite, kaolinite,
and beidellite. Almost all clay minerals were
characterized by an abundance of surface charges
(Kumar et al., 2016; Calarge et al., 2013). Clay
minerals presently used were endowed with -2
charges, unless otherwise noted. As suggested
(Wang et al., 2017), metal ions were adsorbed on the
external surfaces of clay minerals. In line with
previous literatures (Tian et al., 2015; Jia et al.,
2018), the hexagonal rings of silica surfaces along
with adsorbed metal ions were selected as the high-
level region that was handled at the B3LYP/6-
31+G(d,p) level of theory, while the rest of models
were treated as the low-level region and simulated
by the B3LYP/3-21G method. Owing to the
significance of relativistic effects, the inner and
valence electrons of Cs were described by the
LanL2DZ effective core potential and LanL2DZ
basis set, respectively.
3 RESULTS AND DISCUSSION
3.1 K
+
adsorption onto
Montmorillonite Surfaces
Top (upper panel) and side (lower panel) views of
models are shown, and Si, Al, Mg, O and H are
presented in blue, pink, yellow, red and white balls,
respectively.
Figure 1: Models of montmorillonite (MMT), beidellite
(BEI) and kaolinite (KAO).
With the increase of negative charges in
montmorillonite caused by more Mg
2+
/Al
3+
substitutions, the distances of K
+
to the adjacent
surface-O atoms (O
S
) showed a gradual reduction,
consistent with the previous results (Wang et al.,
2017; Tian et al., 2015). For (the number of
negative charges) = 1, 2, 3, the distances of K
+
to
three closet O
S
atoms were averaged at 2.734, 2.684
and 2.657 Å, suggesting that there were stronger
interactions between metal ions and clay surfaces
due to the increase of negative charges. The
interaction energies between K
+
and the
montmorillonite models were calculated to be -522.5,
-739.6 and -976.8 kJ/mol, respectively, and the
adsorption performances of metal ions were
substantially enhanced at clay surfaces with more
negative charges (Wang et al., 2017; Tian et al.,
2015). It demonstrated that the quantity of negative
charges plays a critical role during the adsorption of
metal ions (Wang et al., 2017; Li et al., 2017).
Figure 2 depicted the adsorption configurations
of one, two and three K
+
ions onto the surfaces of
montmorillonite with two Mg
2+
/Al
3+
substitutions
(Calarge et al., 2013). Compared with the first K
+
ion (n = 1), the adsorption strengths of subsequent
K
+
ions considerably reduced, and the interactions
Computer Simulations to Predict the Adsorption and Cation Exchange at Mineral Surfaces
315