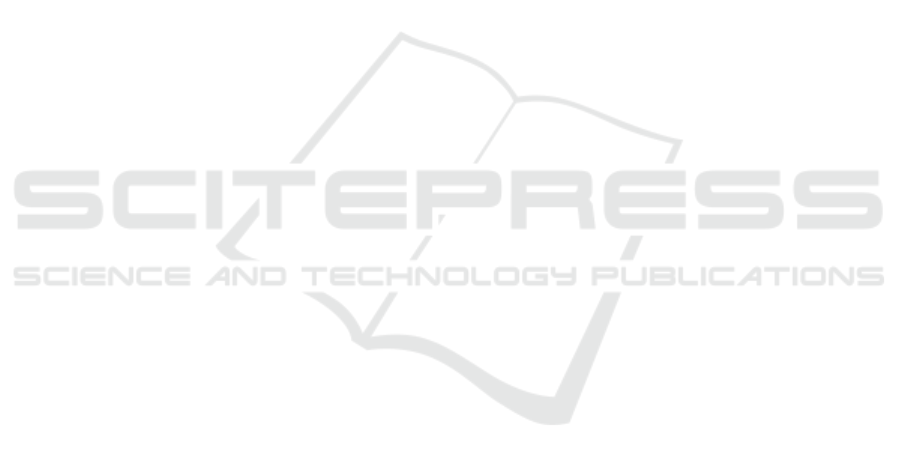
Attapulgite-based Adsorbent for Mercury (II) Removal in
Aqueous Solution
M Li
1, *
, B Li
1
, Y Wang
1
, R J Wang
1
and Q D Zeng
2,3
1
Department of Environmental Science & Engineering, North China Electric Power
University, Baoding, China
2
National Center for International Research on Green Optoelectronics, South China
Normal University, Guangzhou, China
3
Guangdong Provincial Key Laboratory of Optical Information Materials and
Technology and Institute of Electronic Paper Displays, South China Academy of
Advanced Optoelectronics, South China Normal University, Guangzhou, China
Corresponding author and e-mail: M Li, ming2999@126.com
Abstract. A new attapulgite-based adsorbent P-ATP was successfully prepared to remove
mercury (II) from aqueous solutions via grafting reactions. The structures of the products
were characterized by Fourier Transform Infrared Spectra (FTIR) and scanning electron
microscope (SEM) analysis. The adsorption process was investigated by the batch adsorption
experiments. The influence factors including pH, temperature, and contact time were all
discussed. To further understand the adsorption process between adsorbents and the heavy
metals, pseudo-second-order kinetic model was introduced, and Langmuir adsorption
isotherm model was also used to describe the adsorption process. The adsorption capacity of
Hg (II) on P-ATP was calculated to be as high as 181.16 mg/g. Furthermore, the adsorbent
could be reused after 7 times of adsorption-desorption cycles.
1. Introduction
In recent years, heavy metal pollution in water environments becomes much more serious than ever
before [1, 2]. Kinds of heavy metal ions are discharged into rivers, lakes, oceans, and underground
waters with the rapid development of economy and modern industry. Among the released heavy
metals, mercury is considered to be one of the most toxic elements due to its great harm to the human
body. Furthermore, mercury could be enriched in the creatures by the food chain, thus, it makes the
creatures at the top of the food chain suffer more damage [3]. Moreover, excess mercury may cause
dysfunction of liver, kidney, brain tissue, and other serious problems [4].
Up to now, a great number of chemical and physical techniques have been used to eliminate
mercury in water, such as chemical precipitation, ion-exchange, membrane filtration, electrochemical
treatment. However, the above methods have obvious disadvantages: either operational complexity
or high cost [5]. Therefore, adsorption technique becomes a useful and effective approach for the
elimination of mercury in water [6, 7]. Attapulgite (ATP) is a kind of hydrated magnesium aluminum
silicate mineral, which is very cheap and easy to be chemically modified via reactions with hydroxyl
558
Li, M., Li, B., Wang, Y., Wang, R. and Zeng, Q.
Attapulgite-based Adsorbent for Mercury (Ii) Removal in Aqueous Solution.
In Proceedings of the International Workshop on Materials, Chemistry and Engineering (IWMCE 2018), pages 558-564
ISBN: 978-989-758-346-9
Copyright © 2018 by SCITEPRESS – Science and Technology Publications, Lda. All rights reserved