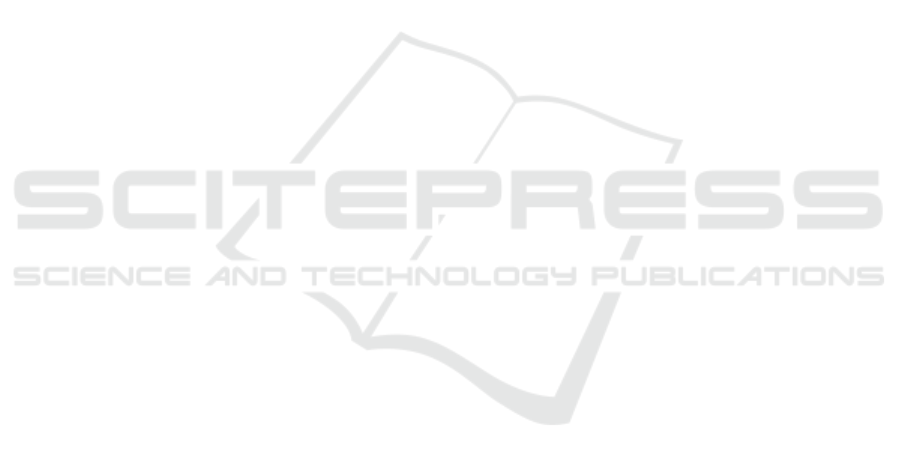
carbons exhibit a nearly rectangular shape, which is typical behaviour for supercapacitors [36,37].
Compared to all other carbon samples, NPC-1000 shows the largest CV area and a more rectangular
shape. The GC curves (Figrue 5b) at a current density of 0.1 A g
-1
are symmetric, and NPC-1000
possesses the longest discharge time. The specific capacitances of the six nanoporous carbons are
listed in Table 1, these values were calculated according to the discharge process. Obviously, NPC-
1000 presents the largest capacitance value of 207.7 F g
-1
compared to other porous carbons. This
result presumably arises from both the higher percentage of graphitic carbons and the suitable
hierarchical micro/meso/macropores structure of NPC-1000, which are advantageous for electrical
conductivity and quick diffusion of electrolyte to the electrode [38-40]. It must be noted that although
NPC-T-1000 possesses the largest value of surface area, its capacitance is only 181.5 F g
-1
, which is
even lower than the values of NPC-800 and NPC-900. The phenomenon reveals that capacitance is
not in direct proportion to the specific surface area, there is only part of micropores are in effective
use when the charge is transfered.
Figure 5c shows the electrochemical impedance spectroscopy, providing available details about
the frequency responses of six ZIF-derived nanoporous carbons as supercapacitor electrode materials.
In the high frequency region, the Nyquist plots exhibit depressed semicircles for the carbons (see the
inset of Figrue 5c), which is related to the charge transport resistance between the electrode and
electrolyte [41]. NPC-1000 shows a smallest crossing value of the Z’ axis, which indicates that NPC-
1000 has lower interfacial contact resistance, because NPC-1000 possesses a hierarchical pore
structure, which is favourable for electrolyte ion migration. In the low frequency region, the larger
the curve slope, the better the capacitive behaviour. A vertical curve indicates ideal capacitive
behaviour and a fast transportation of the electrolyte ions in the nanopores of the carbon materials
[42]. A shift of the vertical curve could result from the Warburg resistance for the electrolyte ions.
Obviously, the EIS curve for NPC-1000 shows the largest value of curve slope, i.e., the lowest
Warburg resistance, which demonstrates that NPC-1000 possesses the best electrical properties
among the nanoporous carbons to act as an electrode material. The specific capacitances of six ZIF-
derived nanoporous carbons at different current densities are presented in Figure 5d. Obviously,
NPC-1000 shows the best capacitive performance. Detailedly, NPC-1000 shows the highest
capacitance of 207.7 F g
-1
at 0.1 A g
-1
. Moreover, the capacitance of NPC-1000 still keep 66.5%
when increasing the current density from 0.1 to 10A g
-1
. In comparison, NPC-T-1000 achieves lower
values of 181.5 F g
-1
and 60.1% under the same test conditions. Obviously, NPC-1000 achieves the
largest retention among the six nanoporous carbon samples.
To further study the electrochemical performance of NPC-1000, we performed a series of
capacitive measurements at different current densities and different scan rates (Figure 6). Figure 6a
exhibits the CV curves of NPC-1000 at different scan rates of 5, 10, 25, 50, 100 and 200 mV s
-1
, all
of them are in typical rectangular shape. At 200 mV s
-1
, the CV curve suffers from some slight
distortion, which may result from the limited mass transfer or ion transport [43]. As shown in Figure
6b, the triangle charge/discharge curves of NPC-1000 exhibit almost linear and symmetrical shapes
at different current densities ranging from 0.1 to 10 A g
-1
, indicating that the NPC-1000 electrode
possesses good electrochemical reversibility [44]. The capacitance of NPC-1000 reaches 207.7 F g
-1
at 0.1 A g
-1
. It must be noted that this value is much larger than the ones of ZIF-8-derived nanoporous
carbons using traditional carbonization method, like Z-800 derived from direct carbonization of ZIF-
8 carbonized at 800 °C (130F g
-1
at a scan rate of 50 mV s
-1
) and Z-1000 at 1000 °C (112F g
-1
at a
scan rate of 50 mV s
-1
) [31]. In addition, it was investigated and shown in Figure 6c for long-term
cycle stability of NPC-1000 at a current density of 5 A g
-1
. The capacitance of NPC-1000 keeps
almost unchanged and only 4.7% of capacitance is lost after 1000 cycles, indicating that the material
structures of NPC-1000 are very stable in the charge and discharge process.
IWMCE 2018 - International Workshop on Materials, Chemistry and Engineering
578