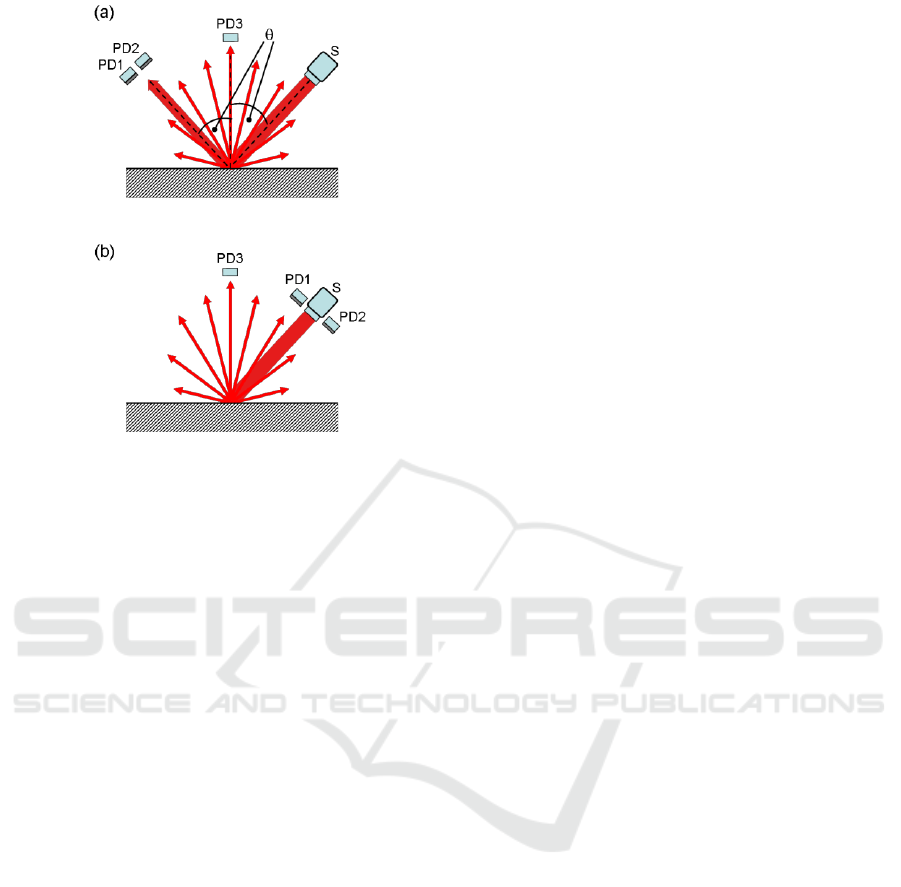
Figure 2: Sketch of the two considered geometries. (a) The
mirror-like configuration exploits the Snell law to detect
the forward scattered light. (b) The backscattering
configuration works with backward diffusion. For both the
configurations, a third photodiode can be added to detect
the unpolarized scattering.
Moreover, the presence of a third photodetector,
collecting the unpolarized scattering to be used as a
further measurement to discriminate between
different surfaces is evaluated. Its position could be
varied as well, but as a reference position we will
consider the direction perpendicular to the surface
normal.
The geometrical arrangements are resumed in
the sketch of fig.2.
4 RESULTS AND DISCUSSION
For both the geometries, the characterization of the
sensor is divided into two parts: a preliminary
measurements is done by varying the incidence
angle of the beam, to check the dependence of the
PCR on the source orientation (Videen, 1992). The
polarization of the source is varied as well, in order
to further improve the system sensitivity to different
conditions, exploiting the polarization dependence
of the Fresnel coefficients. Then, once optimized the
geometry, the detection of the polarization
components and the calculation of the suitable
parameters are performed on asphalt samples.
In all the presented measurements the source has
a power of 18mW and it is at a distance of about
30cm from the surface. We stress that the employed
values can obviously be scaled due to the peculiar
application, but at the moment they try to reproduce
a possible situation when mounting the device on a
moving vehicle or on a road side. Several conditions
have been considered. The safe condition is
indicated by the values provided by dry asphalt,
corresponding to the reference state. Then other
surfaces have been prepared corresponding to
different atmospheric or road conditions, linked to
different level of hazard: wet asphalt, surface
covered by a water layer and the presence of an ice
layer.
4.1 Mirror-like Configuration
For this configuration the source and the detectors
are mounted on a goniometric system ensuring that
incidence and detection angle are equal. Incidence
angle have been varied between 20° and 60° with
respect to the surface normal. Fig. 3 shows the PCR
versus incidence angle for some of the surface
conditions, when the source radiation is unpolarized
or horizontally/vertically polarized.
As it can be seen, both the angle dependence
and the absolute value of PCR are strongly
dependent on polarization. For unpolarized or
horizontally polarized radiation the curves results
superposed, for almost all the angular range. On the
other hand, vertical polarized light allows the
discrimination of different conditions, in particular,
between 50° and 60°. Moreover, for 50° the dry
asphalt (safe) case has the highest value of PCR,
thus it is well distinguishable from the other
conditions. For these reasons, we choose θ=50° to
complete the characterization.
At this angle, we calculate the PCR and plot it
versus the unpolarized scattering. We performed the
measurements a number of times to include the
statistical errors of both measurements, also
considering several samples for each conditions.
Thus, we obtained dispersion graphs on the
parameter space for all the road condition, as
reported in fig.4. In this way, the separation of the
clouds allows finding portions of the parameter
space associated to one state, while clouds
superpositions indicate the possibility of obtaining a
measurement with a certain error percentage.
Fig.4(a) shows a good separation between the
states but for the couple dry-ice, for which we
calculate an error of about 10%. This represents a
problem because the system is interpreting a
potentially dangerous condition as a safe one (or
vice versa). For this reason we checked if it exists
another way to represent the different road
A Sensor for the Optical Detection of Dangerous Road Condition
285